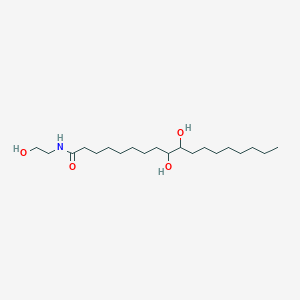
9,10-Dihydroxy-N-(2-hydroxyethyl)octadecanamide
- Click on QUICK INQUIRY to receive a quote from our team of experts.
- With the quality product at a COMPETITIVE price, you can focus more on your research.
Overview
Description
9,10-Dihydroxy-N-(2-hydroxyethyl)octadecanamide is a chemical compound with the molecular formula C20H41NO4 It is a derivative of octadecanamide, featuring hydroxyl groups at the 9th and 10th positions and an additional hydroxyethyl group attached to the nitrogen atom
Preparation Methods
Synthetic Routes and Reaction Conditions
The synthesis of 9,10-Dihydroxy-N-(2-hydroxyethyl)octadecanamide typically involves the hydroxylation of octadecanamide followed by the introduction of a hydroxyethyl group. One common method includes the use of hydrogen peroxide as an oxidizing agent to introduce hydroxyl groups at the 9th and 10th positions of octadecanamide. The reaction is usually carried out under controlled temperature and pH conditions to ensure selective hydroxylation.
Industrial Production Methods
In an industrial setting, the production of this compound may involve large-scale hydroxylation processes using continuous flow reactors. These reactors allow for precise control over reaction parameters, ensuring high yield and purity of the final product. The hydroxyethylation step can be achieved using ethylene oxide or ethylene glycol under basic conditions.
Chemical Reactions Analysis
Types of Reactions
9,10-Dihydroxy-N-(2-hydroxyethyl)octadecanamide undergoes various chemical reactions, including:
Oxidation: The hydroxyl groups can be further oxidized to form carbonyl or carboxyl groups.
Reduction: The compound can be reduced to remove the hydroxyl groups, reverting to octadecanamide.
Substitution: The hydroxyethyl group can be substituted with other functional groups through nucleophilic substitution reactions.
Common Reagents and Conditions
Oxidation: Common oxidizing agents include potassium permanganate and chromium trioxide.
Reduction: Reducing agents such as lithium aluminum hydride or sodium borohydride are used.
Substitution: Nucleophiles like amines or thiols can be used under basic or acidic conditions.
Major Products Formed
Oxidation: Formation of 9,10-diketooctadecanamide or 9,10-dicarboxyoctadecanamide.
Reduction: Formation of octadecanamide.
Substitution: Formation of various substituted octadecanamides depending on the nucleophile used.
Scientific Research Applications
9,10-Dihydroxy-N-(2-hydroxyethyl)octadecanamide has several applications in scientific research:
Chemistry: Used as a precursor in the synthesis of complex organic molecules and polymers.
Biology: Studied for its potential role in cell signaling and membrane interactions.
Medicine: Investigated for its potential therapeutic effects, including anti-inflammatory and antimicrobial properties.
Industry: Utilized in the formulation of surfactants, lubricants, and emulsifiers.
Mechanism of Action
The mechanism of action of 9,10-Dihydroxy-N-(2-hydroxyethyl)octadecanamide involves its interaction with cellular membranes and proteins. The hydroxyl groups allow for hydrogen bonding with membrane lipids and proteins, potentially altering membrane fluidity and protein function. The hydroxyethyl group may also participate in specific biochemical pathways, influencing cellular processes.
Comparison with Similar Compounds
Similar Compounds
Octadecanamide: Lacks the hydroxyl and hydroxyethyl groups, resulting in different chemical properties and applications.
9,10-Dihydroxyoctadecanamide: Similar structure but lacks the hydroxyethyl group, affecting its solubility and reactivity.
N-(2-Hydroxyethyl)octadecanamide: Lacks the hydroxyl groups at the 9th and 10th positions, impacting its chemical behavior.
Uniqueness
9,10-Dihydroxy-N-(2-hydroxyethyl)octadecanamide is unique due to the presence of both hydroxyl and hydroxyethyl groups, which confer distinct chemical properties and potential applications. Its ability to undergo various chemical reactions and interact with biological membranes makes it a versatile compound in scientific research.
Properties
CAS No. |
656241-61-1 |
---|---|
Molecular Formula |
C20H41NO4 |
Molecular Weight |
359.5 g/mol |
IUPAC Name |
9,10-dihydroxy-N-(2-hydroxyethyl)octadecanamide |
InChI |
InChI=1S/C20H41NO4/c1-2-3-4-5-7-10-13-18(23)19(24)14-11-8-6-9-12-15-20(25)21-16-17-22/h18-19,22-24H,2-17H2,1H3,(H,21,25) |
InChI Key |
XUTGMKCKJJYMGA-UHFFFAOYSA-N |
Canonical SMILES |
CCCCCCCCC(C(CCCCCCCC(=O)NCCO)O)O |
Origin of Product |
United States |
Disclaimer and Information on In-Vitro Research Products
Please be aware that all articles and product information presented on BenchChem are intended solely for informational purposes. The products available for purchase on BenchChem are specifically designed for in-vitro studies, which are conducted outside of living organisms. In-vitro studies, derived from the Latin term "in glass," involve experiments performed in controlled laboratory settings using cells or tissues. It is important to note that these products are not categorized as medicines or drugs, and they have not received approval from the FDA for the prevention, treatment, or cure of any medical condition, ailment, or disease. We must emphasize that any form of bodily introduction of these products into humans or animals is strictly prohibited by law. It is essential to adhere to these guidelines to ensure compliance with legal and ethical standards in research and experimentation.