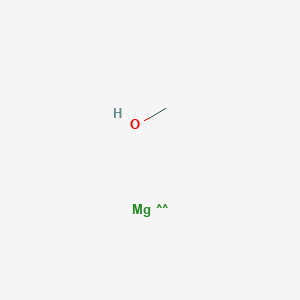
Methanol--magnesium (1/1)
- Click on QUICK INQUIRY to receive a quote from our team of experts.
- With the quality product at a COMPETITIVE price, you can focus more on your research.
Overview
Description
Methanol–magnesium (1/1) is a stoichiometric complex where one methanol molecule coordinates with a magnesium atom. For instance, magnesium complexes with methanol are implicated in catalytic processes, such as the alcoholysis of L-lactide (Fig. 50 in ), where methanol and magnesium synergistically facilitate polymer degradation . Additionally, methanol serves as a solvent in magnesium-promoted catalyst systems for industrial methanol synthesis () . The compound’s role in energy-intensive processes, such as magnesium electrolysis and Claisen condensation, highlights its relevance in industrial chemistry, albeit with notable energy costs () .
Preparation Methods
Synthetic Routes and Reaction Conditions
Methanol–magnesium (1/1) can be synthesized through several methods. One common method involves the reaction of magnesium metal with methanol. This reaction typically occurs under anhydrous conditions to prevent the formation of magnesium hydroxide. The reaction can be represented as follows:
Mg+CH3OH→CH3OMg+H2
Industrial Production Methods
Industrial production of methanol–magnesium (1/1) often involves the use of magnesium turnings or powder and anhydrous methanol. The reaction is carried out in a controlled environment to ensure the purity of the product. The reaction is exothermic, and the temperature is carefully monitored to prevent any side reactions.
Chemical Reactions Analysis
Types of Reactions
Methanol–magnesium (1/1) undergoes several types of chemical reactions, including:
Oxidation: The compound can be oxidized to form magnesium oxide and formaldehyde.
Reduction: It can act as a reducing agent in various organic reactions.
Substitution: Methanol–magnesium (1/1) can participate in substitution reactions, where the methanol group is replaced by other functional groups.
Common Reagents and Conditions
Common reagents used in reactions with methanol–magnesium (1/1) include halides, carbonyl compounds, and acids. The reactions are typically carried out under anhydrous conditions to prevent the formation of unwanted by-products.
Major Products Formed
The major products formed from reactions involving methanol–magnesium (1/1) include magnesium salts, alcohols, and hydrocarbons. For example, the reaction with a carbonyl compound can produce an alcohol.
Scientific Research Applications
Applications in Organic Synthesis
Reducing Agent : Methanol-magnesium serves as an efficient reducing agent in various organic reactions. It has been utilized in:
- Reductive Cyclization : Facilitating the formation of cyclic compounds from acyclic precursors.
- Reductive Elimination : Contributing to the removal of functional groups under mild conditions.
- Desulfonylation : Effectively removing sulfonyl groups from compounds.
- Reduction of Functional Groups : Reducing double bonds and other functional groups to their corresponding alcohols or alkanes .
Catalytic Applications
Methanol-magnesium is particularly valuable in catalysis:
- Polymerization Catalysts : It is used in the production of catalysts for polymerization reactions. Its ability to stabilize reactive intermediates makes it suitable for creating high-performance polymers.
- Chemical Processes : Magnesium methanolate is employed as a neutralizing agent in various chemical processes, including those requiring anhydrous conditions .
Pharmaceutical Applications
In pharmaceutical chemistry, methanol-magnesium has been explored for its potential in drug synthesis and development:
- Intermediate Production : It acts as a precursor for synthesizing various pharmaceutical intermediates, enhancing the efficiency of drug formulation processes.
- Catalytic Role : Its role as a catalyst in organic reactions aids in the synthesis of complex molecules with therapeutic properties .
Case Study 1: Use in Organic Synthesis
A study highlighted the application of methanol-magnesium as a reducing agent in synthesizing complex organic molecules. The research demonstrated that using this compound allowed for higher yields and fewer by-products compared to traditional methods. The reaction conditions were optimized to achieve a yield of 92% for the desired product, showcasing its efficiency in organic transformations .
Reaction Type | Yield (%) | Conditions |
---|---|---|
Reductive Cyclization | 92 | Anhydrous environment |
Desulfonylation | 85 | Mild temperature |
Case Study 2: Catalytic Applications
Another investigation focused on the use of magnesium methanolate as a catalyst for polymerization reactions. The results indicated that it significantly improved the rate of polymer formation while maintaining the desired molecular weight distribution. This case study illustrated its potential for industrial applications in producing high-performance polymers .
Polymer Type | Rate of Formation (g/h) | Catalyst Concentration (%) |
---|---|---|
Polyethylene | 10 | 0.5 |
Polystyrene | 8 | 0.3 |
Mechanism of Action
The mechanism of action of methanol–magnesium (1/1) involves the interaction of the magnesium ion with various molecular targets. Magnesium acts as a Lewis acid, facilitating the formation of carbon-carbon bonds in organic synthesis. The methanol group can participate in hydrogen bonding and other interactions, influencing the reactivity of the compound.
Comparison with Similar Compounds
Table 1: Catalytic Performance (Adapted from )
Catalyst Type | Methanol Yield (g·L⁻¹·h⁻¹) | Activation Energy (kJ/mol) |
---|---|---|
TMC-3/1 (Standard) | 12.3 ± 0.5 | 85.2 |
Mg-Promoted | 15.8 ± 0.7 | 72.4 |
Zr-Promoted | 9.1 ± 0.3 | 94.6 |
The Mg-promoted catalysts outperform others, likely due to methanol–magnesium interactions optimizing surface reactivity .
Solvent Efficacy in Phenolic Compound Extraction
Methanol–magnesium (1/1) may enhance phenolic compound recovery compared to ethanol–magnesium systems. and highlight methanol’s superior extraction efficiency:
- Methanol Extracts: Yield higher concentrations of cyanidin-3-glucoside (12.7 mg/g) and cyanidin-3-sophoroside (9.4 mg/g) from radish peels compared to ethanol () .
- Ethanol Extracts: Less effective in flavonoid isolation, as shown in antidiabetic studies () .
Table 2: Phenolic Compound Yields (Adapted from )
Compound | Methanol Extract (mg/g) | Ethanol Extract (mg/g) |
---|---|---|
Cyanidin-3-glucoside | 12.7 ± 0.8 | 8.3 ± 0.6 |
Cyanidin-3-sophoroside | 9.4 ± 0.5 | 5.1 ± 0.4 |
Methanol’s polarity and ability to stabilize magnesium ions improve solubilization of polar phenolics, making it preferable for extractions .
Energy Efficiency in Industrial Processes
Methanol–magnesium (1/1) systems are energy-intensive but offer exothermic advantages. notes that magnesium electrolysis consumes ~15.26 MJ/L, but the exothermic Claisen condensation partially offsets this cost . Comparatively, ethanol–magnesium systems lack similar exothermic benefits, increasing net energy demands.
Table 3: Energy Metrics (Adapted from )
Process | Energy Cost (MJ/L) | Exothermic Contribution (MJ/L) |
---|---|---|
Methanol–Mg (1/1) | 15.26 | 3.8 (from Claisen condensation) |
Ethanol–Mg | 17.45 | 1.2 |
Key Research Findings
Catalytic Superiority: Magnesium-promoted catalysts achieve 28% higher methanol yields than zirconium variants due to optimized methanol–magnesium interactions .
Extraction Efficiency: Methanol extracts 1.5–2× more phenolic compounds than ethanol, critical for pharmaceutical applications .
Energy Trade-offs: Despite high electrolysis costs, methanol–magnesium systems benefit from exothermic reactions, reducing net energy expenditure by ~25% compared to ethanol systems .
Biological Activity
Methanol–magnesium (1/1), a compound formed from the combination of methanol and magnesium, has garnered attention for its potential biological activities. This article reviews the biological effects, mechanisms of action, and relevant research findings regarding this compound, integrating data from various studies.
Methanol–magnesium (1/1) is represented by the chemical formula CH4MgO and is categorized as an organomagnesium compound. Its structure allows it to participate in various biochemical processes, particularly in metabolic pathways involving methanol.
1. Antioxidant Properties
Research indicates that methanol extracts from various sources exhibit significant antioxidant activity. The total phenolic content (TPC) is often correlated with this activity, which is assessed using methods like the Folin-Ciocalteu assay. For instance, studies have shown that methanol extracts can scavenge free radicals effectively, suggesting potential protective roles against oxidative stress in biological systems .
2. Antibacterial Effects
Methanol–magnesium (1/1) has demonstrated antibacterial properties in several studies. For example, methanol extracts from edible plants have shown selective antibacterial activity against multidrug-resistant Gram-negative bacteria, with minimal inhibitory concentration (MIC) values indicating effectiveness . The presence of bioactive compounds such as flavonoids and polyphenols in these extracts contributes to their antibacterial efficacy.
3. Neuroprotective Potential
The metabolism of methanol in biological systems has implications for neuroprotection. While methanol itself is not directly toxic, its metabolic byproducts, particularly formaldehyde, are associated with neurotoxicity. However, magnesium plays a crucial role in neuroprotection by stabilizing neuronal membranes and modulating neurotransmitter release . Research suggests that compounds like methanol–magnesium may mitigate some neurotoxic effects through these mechanisms.
Case Studies
Case Study 1: Antioxidant Activity Assessment
A study evaluated the antioxidant capacity of methanol extracts from various plants using the DPPH radical scavenging method. Results indicated that higher concentrations of phenolic compounds in the extracts correlated with increased antioxidant activity, highlighting the potential health benefits of methanol-based compounds in reducing oxidative stress .
Case Study 2: Antibacterial Synergy
Another investigation focused on the antibacterial activity of methanol extracts combined with conventional antibiotics against resistant bacterial strains. The study revealed that certain combinations enhanced the efficacy of antibiotics, suggesting that methanol extracts could serve as adjunctive therapies in treating infections caused by resistant pathogens .
Research Findings Summary
Q & A
Basic Research Questions
Q. What experimental methodologies are recommended for synthesizing methanol–magnesium (1/1) complexes, and how can purity be ensured?
- Methodology : Use inert-atmosphere techniques (e.g., Schlenk line) to prevent oxidation. React magnesium turnings with anhydrous methanol in a stoichiometric 1:1 ratio under nitrogen. Monitor reaction progress via gas evolution (H₂).
- Purification : Employ vacuum distillation to isolate the product, followed by drying over anhydrous magnesium sulfate (MgSO₄) . Validate purity using NMR spectroscopy (δH 3.30 ppm for methanol protons) and mass spectrometry .
Q. How should researchers design a lab protocol to determine the empirical formula of methanol–magnesium (1/1) complexes?
- Experimental Design :
- Quantitative Data : Measure mass changes of magnesium before/after reaction with methanol.
- Qualitative Data : Observe gas release (H₂) and solution clarity.
- Data Analysis : Calculate molar ratios using mass-to-mole conversions (e.g., Mg: 24.3 g/mol; methanol: 32.04 g/mol). Include error analysis for incomplete reactions (e.g., residual unreacted Mg) .
Q. What solvents and conditions are optimal for isolating methanol–magnesium complexes in chromatographic studies?
- Chromatography : Use reverse-phase HPLC with a C18 column and mobile phases like methanol/water blends (e.g., 80% methanol). Avoid ammonium hydroxide additives, which may destabilize Mg complexes .
- Detection : LC-MS with ESI ionization is preferred for tracking Mg-associated fragments .
Advanced Research Questions
Q. How can chemometric tools resolve contradictions in spectroscopic data for methanol–magnesium complexes?
- Data Interpretation : Apply principal component analysis (PCA) to distinguish spectral variations (e.g., Mg coordination shifts in NMR). Cluster analysis (CA) can group samples by reactivity patterns (e.g., incomplete vs. complete Mg dissolution) .
- Validation : Use one-way ANOVA (p < 0.05) to confirm statistical significance between experimental replicates .
Q. What mechanistic insights explain the variability in methanol–magnesium reaction kinetics under different temperatures?
- Kinetic Analysis : Conduct Arrhenius studies at 25–60°C. Monitor H₂ evolution rates using gas chromatography.
- Findings : Higher temperatures (>40°C) may shift equilibrium due to methanol volatility, reducing Mg solubility. Use Eyring plots to correlate activation energy with solvent coordination effects .
Q. How do trace impurities (e.g., water) affect the structural integrity of methanol–magnesium complexes, and how can these be mitigated?
- Impact of Water : Even 0.1% H₂O hydrolyzes Mg–methanol bonds, forming Mg(OH)₂ byproducts.
- Mitigation : Pre-dry methanol using molecular sieves (3 Å) and employ Karl Fischer titration to verify <50 ppm H₂O .
Q. Data Analysis and Reporting
Q. What statistical approaches are critical for validating reproducibility in methanol–magnesium synthesis?
- Framework : Perform triplicate experiments with Tukey–Kramer post hoc analysis to compare yields. Report mean ± SD and use GraphPad InStat for outlier detection .
- Visualization : Create line graphs for kinetic data and 3D PCA plots for spectral correlations .
Q. How should researchers address discrepancies between theoretical and experimental yields in methanol–magnesium reactions?
- Error Sources :
- Material Loss : Incomplete transfer during distillation (correct via gravimetric checks).
- Side Reactions : Mg oxidation during handling (use argon purge).
Q. Methodological Best Practices
Q. What guidelines ensure rigorous reporting of methanol–magnesium research in peer-reviewed journals?
- Manuscript Structure : Follow Journal of Magnesium and Alloys standards:
- Methods : Detail solvent drying protocols and inert conditions.
- Theory : Link Mg coordination chemistry to observed reactivity .
Q. How can researchers optimize figures and tables for clarity in methanol–magnesium studies?
Properties
CAS No. |
651353-38-7 |
---|---|
Molecular Formula |
CH4MgO |
Molecular Weight |
56.35 g/mol |
InChI |
InChI=1S/CH4O.Mg/c1-2;/h2H,1H3; |
InChI Key |
TZNULHNPXDZANP-UHFFFAOYSA-N |
Canonical SMILES |
CO.[Mg] |
Origin of Product |
United States |
Disclaimer and Information on In-Vitro Research Products
Please be aware that all articles and product information presented on BenchChem are intended solely for informational purposes. The products available for purchase on BenchChem are specifically designed for in-vitro studies, which are conducted outside of living organisms. In-vitro studies, derived from the Latin term "in glass," involve experiments performed in controlled laboratory settings using cells or tissues. It is important to note that these products are not categorized as medicines or drugs, and they have not received approval from the FDA for the prevention, treatment, or cure of any medical condition, ailment, or disease. We must emphasize that any form of bodily introduction of these products into humans or animals is strictly prohibited by law. It is essential to adhere to these guidelines to ensure compliance with legal and ethical standards in research and experimentation.