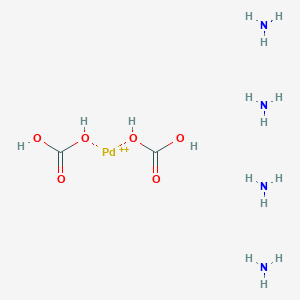
Tetraamminepalladium(II)hydrogencarbonate
- Click on QUICK INQUIRY to receive a quote from our team of experts.
- With the quality product at a COMPETITIVE price, you can focus more on your research.
Overview
Description
Tetraamminepalladium (II) hydrogen carbonate is a coordination complex of palladium. It is a compound with the molecular formula C₂H₁₄N₄O₆Pd and a molecular weight of 296.58 g/mol . This compound is known for its applications in various fields, including catalysis and material science.
Preparation Methods
Synthetic Routes and Reaction Conditions
Tetraamminepalladium (II) hydrogen carbonate can be synthesized by reacting palladium chloride with ammonia in the presence of carbon dioxide. The reaction typically involves dissolving palladium chloride in water, followed by the addition of ammonia and carbon dioxide. The resulting solution is then allowed to crystallize, yielding tetraamminepalladium (II) hydrogen carbonate .
Industrial Production Methods
Industrial production of tetraamminepalladium (II) hydrogen carbonate follows a similar synthetic route but on a larger scale. The process involves careful control of reaction conditions, such as temperature and pH, to ensure high yield and purity of the final product .
Chemical Reactions Analysis
Types of Reactions
Tetraamminepalladium (II) hydrogen carbonate undergoes various types of chemical reactions, including:
Oxidation: The compound can be oxidized to form palladium oxide.
Reduction: It can be reduced to metallic palladium.
Substitution: The ammonia ligands can be substituted with other ligands, such as phosphines or amines.
Common Reagents and Conditions
Oxidation: Common oxidizing agents include oxygen and hydrogen peroxide.
Reduction: Reducing agents such as hydrogen gas or sodium borohydride are used.
Substitution: Ligand substitution reactions often involve the use of phosphines or amines under mild conditions.
Major Products
Oxidation: Palladium oxide.
Reduction: Metallic palladium.
Substitution: Various palladium complexes with different ligands.
Scientific Research Applications
Tetraamminepalladium (II) hydrogen carbonate has numerous applications in scientific research:
Mechanism of Action
The mechanism of action of tetraamminepalladium (II) hydrogen carbonate involves its ability to form strong complexes with both inorganic and organic ligands. Palladium ions can disturb cellular equilibria, replace other essential ions, and interact with functional groups of macromolecules, such as proteins or DNA . This interaction can lead to various biological effects, including the inhibition of enzyme activity and disruption of cellular processes.
Comparison with Similar Compounds
Similar Compounds
- Tetraammineplatinum (II) hydrogen carbonate
- Tetraamminecopper (II) hydrogen carbonate
- Tetraamminecobalt (III) hydrogen carbonate
Uniqueness
Tetraamminepalladium (II) hydrogen carbonate is unique due to its specific coordination chemistry and the ability of palladium to participate in a wide range of catalytic reactions. Compared to similar compounds, it offers distinct advantages in terms of reactivity and versatility in catalysis .
Biological Activity
Tetraamminepalladium(II)hydrogencarbonate, with the chemical formula [Pd(NH3)4(HCO3)2], is a palladium complex that has garnered attention for its potential biological applications, particularly in the fields of medicine and environmental science. This article explores its biological activity, including its synthesis, mechanisms of action, and relevant case studies.
This compound is synthesized from dichlorodiamminepalladium(II) through a reaction with sodium bicarbonate in an ammonia solution. The general reaction can be summarized as follows:
This method yields a high purity product (>99.95%) and is efficient in avoiding toxic byproducts such as chloride ions .
2.1 Antimicrobial Properties
Research indicates that palladium complexes, including this compound, exhibit significant antimicrobial activity. A study demonstrated that palladium(II) complexes possess antibacterial properties against various bacterial strains, including both Gram-positive (e.g., Staphylococcus aureus) and Gram-negative bacteria . The mechanism of action is believed to involve the disruption of bacterial cell membranes and interference with metabolic processes.
2.2 Cytotoxicity and Anticancer Potential
This compound has shown promise in anticancer research. In vitro studies have reported that palladium complexes can induce apoptosis in cancer cells through the generation of reactive oxygen species (ROS) and the activation of stress response pathways . The compound's interaction with DNA has also been documented, suggesting potential mutagenic effects that could be leveraged for targeted cancer therapies .
Case Study 1: Antibacterial Efficacy
A recent study investigated the antibacterial efficacy of this compound against Escherichia coli and Staphylococcus aureus. The results indicated a significant reduction in bacterial viability at concentrations as low as 10 µM, highlighting its potential as a therapeutic agent in treating bacterial infections .
Case Study 2: Cytotoxic Effects on Cancer Cells
In another study, this compound was tested on human breast cancer cell lines (MCF-7). The compound exhibited a dose-dependent cytotoxic effect, with IC50 values indicating effective cell death at concentrations above 20 µM. Flow cytometry analysis revealed that the compound induced apoptosis through mitochondrial pathways .
The biological activity of this compound can be attributed to several mechanisms:
- DNA Interaction : Studies have shown that palladium can bind to DNA, leading to structural changes that may inhibit replication and transcription processes .
- Oxidative Stress Induction : Palladium complexes can generate ROS, which damage cellular components and trigger apoptotic pathways in cancer cells .
- Membrane Disruption : The interaction with bacterial membranes leads to increased permeability and eventual cell lysis .
5. Safety and Toxicology
Despite its promising biological activities, the safety profile of this compound requires careful consideration. Toxicological studies indicate potential nephrotoxic effects at high concentrations, necessitating further research to establish safe dosage levels for therapeutic use .
Summary Table of Biological Activities
Properties
Molecular Formula |
C2H16N4O6Pd+2 |
---|---|
Molecular Weight |
298.59 g/mol |
IUPAC Name |
azane;carbonic acid;palladium(2+) |
InChI |
InChI=1S/2CH2O3.4H3N.Pd/c2*2-1(3)4;;;;;/h2*(H2,2,3,4);4*1H3;/q;;;;;;+2 |
InChI Key |
QYYPTZGPXKUKRM-UHFFFAOYSA-N |
Canonical SMILES |
C(=O)(O)O.C(=O)(O)O.N.N.N.N.[Pd+2] |
Origin of Product |
United States |
Disclaimer and Information on In-Vitro Research Products
Please be aware that all articles and product information presented on BenchChem are intended solely for informational purposes. The products available for purchase on BenchChem are specifically designed for in-vitro studies, which are conducted outside of living organisms. In-vitro studies, derived from the Latin term "in glass," involve experiments performed in controlled laboratory settings using cells or tissues. It is important to note that these products are not categorized as medicines or drugs, and they have not received approval from the FDA for the prevention, treatment, or cure of any medical condition, ailment, or disease. We must emphasize that any form of bodily introduction of these products into humans or animals is strictly prohibited by law. It is essential to adhere to these guidelines to ensure compliance with legal and ethical standards in research and experimentation.