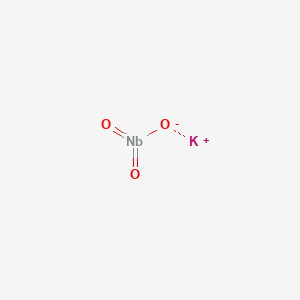
potassium;oxido(dioxo)niobium
- Click on QUICK INQUIRY to receive a quote from our team of experts.
- With the quality product at a COMPETITIVE price, you can focus more on your research.
Overview
Description
Preparation Methods
Synthetic Routes and Reaction Conditions
Potassium niobate can be synthesized through various methods. One common approach is the solvothermal synthesis, where niobium chloride is dissolved in benzyl alcohol, forming octahedral [NbCl₆−xO] complexes through the exchange of chloride ligands. Heating the solution results in polymerization, leading to the formation of nucleation clusters with the ReO₃ type structure, which eventually grow into potassium niobate nanoparticles .
Another method involves the use of niobium hydroxide as a niobium source and oxalate as a complexing agent. The process includes dissolving niobium hydroxide powder in an oxalate solution, followed by the addition of potassium carbonate, oxide, or hydroxide. The solution is then dried, precalcined, and calcined to obtain potassium niobate powder .
Industrial Production Methods
Industrial production of potassium niobate often involves high-temperature solid-state reactions. Niobium pentoxide and potassium carbonate are mixed and heated to high temperatures to form potassium niobate. This method is widely used due to its simplicity and scalability .
Chemical Reactions Analysis
Types of Reactions
Potassium niobate undergoes various chemical reactions, including:
Oxidation: Potassium niobate can be oxidized to form higher oxidation state compounds.
Reduction: It can be reduced to lower oxidation state compounds.
Substitution: Potassium niobate can undergo substitution reactions where potassium ions are replaced by other cations.
Common Reagents and Conditions
Common reagents used in these reactions include oxidizing agents like hydrogen peroxide, reducing agents like sodium borohydride, and various cationic salts for substitution reactions. The reactions typically occur under controlled temperature and pH conditions to ensure the desired product formation .
Major Products Formed
The major products formed from these reactions include various niobium oxides and substituted niobates, depending on the specific reagents and conditions used .
Scientific Research Applications
Potassium niobate has a wide range of scientific research applications:
Mechanism of Action
The mechanism by which potassium niobate exerts its effects involves its interaction with various molecular targets and pathways. For example, in catalysis, potassium niobate acts as a catalyst by providing active sites for the reaction to occur, thereby increasing the reaction rate. In biological applications, its nanoparticles can interact with cellular components, facilitating imaging or drug delivery .
Comparison with Similar Compounds
Similar Compounds
Similar compounds to potassium niobate include:
Sodium niobate (NaNbO₃): Similar in structure and properties but with sodium instead of potassium.
Lithium niobate (LiNbO₃): Known for its excellent piezoelectric and electro-optic properties.
Calcium niobate (CaNb₂O₆): Used in various electronic applications.
Uniqueness
Potassium niobate is unique due to its large nonlinear optical coefficients, making it ideal for applications in wavelength conversion and optical parametric oscillators. Its lead-free nature also makes it an environmentally friendly alternative to lead-based piezoelectric materials .
Properties
Molecular Formula |
KNbO3 |
---|---|
Molecular Weight |
180.003 g/mol |
IUPAC Name |
potassium;oxido(dioxo)niobium |
InChI |
InChI=1S/K.Nb.3O/q+1;;;;-1 |
InChI Key |
UKDIAJWKFXFVFG-UHFFFAOYSA-N |
Canonical SMILES |
[O-][Nb](=O)=O.[K+] |
Origin of Product |
United States |
Disclaimer and Information on In-Vitro Research Products
Please be aware that all articles and product information presented on BenchChem are intended solely for informational purposes. The products available for purchase on BenchChem are specifically designed for in-vitro studies, which are conducted outside of living organisms. In-vitro studies, derived from the Latin term "in glass," involve experiments performed in controlled laboratory settings using cells or tissues. It is important to note that these products are not categorized as medicines or drugs, and they have not received approval from the FDA for the prevention, treatment, or cure of any medical condition, ailment, or disease. We must emphasize that any form of bodily introduction of these products into humans or animals is strictly prohibited by law. It is essential to adhere to these guidelines to ensure compliance with legal and ethical standards in research and experimentation.