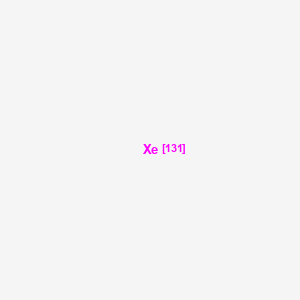
Xenon-131
Overview
Description
Xenon-131 is a stable isotope of the noble gas xenon, which is part of Group 18 on the periodic table. It has an atomic number of 54 and a mass number of 131, consisting of 54 protons and 77 neutrons . This compound is one of the naturally occurring isotopes of xenon and is known for its applications in various scientific fields due to its unique properties.
Preparation Methods
Synthetic Routes and Reaction Conditions: Xenon-131 can be produced through the neutron irradiation of stable xenon isotopes such as xenon-130 or xenon-132. This process involves exposing the target isotope to a flux of neutrons, resulting in the capture of neutrons and the formation of this compound .
Industrial Production Methods: Xenon is typically extracted from the atmosphere through the fractional distillation of liquid air. The process involves cooling air to very low temperatures to liquefy it, followed by the separation of xenon from other gases based on their boiling points . Once xenon is isolated, isotopic enrichment techniques such as gas centrifugation or electromagnetic isotope separation can be used to obtain this compound.
Chemical Reactions Analysis
Types of Reactions: Xenon-131, like other noble gases, is chemically inert under standard conditions. it can form compounds under specific conditions, particularly with highly electronegative elements such as fluorine and oxygen .
Common Reagents and Conditions:
Fluorination: Xenon can react with fluorine gas under controlled conditions to form xenon fluorides such as xenon difluoride (XeF2), xenon tetrafluoride (XeF4), and xenon hexafluoride (XeF6).
Major Products Formed:
Xenon Difluoride (XeF2): Formed by the direct reaction of xenon with fluorine gas.
Xenon Tetrafluoride (XeF4): Formed by the reaction of xenon with an excess of fluorine gas.
Xenon Trioxide (XeO3): Formed by the hydrolysis of xenon hexafluoride.
Scientific Research Applications
Medical Applications
1.1 Anesthetic Properties
Xenon-131 has been studied for its anesthetic properties. Research indicates that it exhibits lower anesthetic potency compared to other xenon isotopes due to its nuclear spin of which affects its interaction with the central nervous system. A study involving mice demonstrated that the loss of righting reflex (a measure of anesthetic potency) was significantly influenced by the nuclear spin of xenon isotopes, with this compound being less potent than Xenon-132 and Xenon-134 .
1.2 Neuroprotection
This compound is also recognized for its neuroprotective effects, particularly in hypoxic-ischemic conditions. It acts by blocking the N-methyl-D-aspartate (NMDA) receptor, preventing calcium overload in neurons, which can lead to cell death. Clinical applications have shown that when combined with hypothermia, this compound enhances neuroprotection during surgeries or after traumatic brain injuries .
1.3 Traceability in Doping Control
Due to its unique properties, this compound has been implicated in doping controls in sports. Its rapid elimination from the body and low solubility make it a candidate for monitoring athletes' exposure to xenon gas during anesthesia . Studies have established that this compound can be reliably detected in blood samples for up to 48 hours post-exposure, which is crucial for effective doping regulations.
Imaging Applications
2.1 Magnetic Resonance Imaging (MRI)
This compound has been utilized as a contrast agent in magnetic resonance imaging, particularly for surface-sensitive imaging of materials such as aerogels. Its quadrupolar interactions allow for distinctive imaging features that are not achievable with other isotopes like Xenon-129 . The ability to distinguish between different densities and hydration levels in aerogels through this compound imaging provides valuable insights into material science.
Material Type | Imaging Technique | Contrast Mechanism |
---|---|---|
Aerogels | MRI | Quadrupolar interactions leading to surface-specific contrast |
Nuclear Safety and Environmental Monitoring
3.1 Detection of Radioactive Isotopes
In nuclear safety, this compound plays a role in monitoring radioactive emissions from fission processes. It is one of the isotopes detected by systems designed to monitor compliance with nuclear test ban treaties (CTBT). The development of advanced spectrometers has improved the detection sensitivity of radioactive xenon isotopes, including this compound, significantly enhancing environmental monitoring capabilities .
Case Studies
4.1 Clinical Study on Anesthetic Potency
A clinical study involving C57BL/6 male mice assessed the anesthetic potency of various xenon isotopes including this compound. The results showed that while all xenon isotopes exhibited anesthetic properties, those with nuclear spin (like this compound) were less potent than their non-spinning counterparts .
4.2 Neuroprotective Effects in Clinical Trials
Clinical trials have demonstrated the effectiveness of this compound in providing neuroprotection during surgeries involving potential ischemic events. The combination therapy using this compound and hypothermia showed promising results in reducing neuronal damage post-surgery .
Mechanism of Action
Xenon-131 exerts its effects primarily through its interactions with other elements and compounds. In medical applications, xenon acts as a neuroprotective and anesthetic agent by binding to the glycine site of the glutamatergic N-methyl-D-aspartate (NMDA) receptor, competitively blocking it. This inhibition prevents the overstimulation of NMDA receptors, thereby reducing calcium influx and protecting neurons from excitotoxic damage .
Comparison with Similar Compounds
Xenon-129: Another stable isotope of xenon with applications in NMR spectroscopy and medical imaging.
Xenon-133: A radioactive isotope used in pulmonary ventilation studies and cerebral blood flow assessment.
Uniqueness of Xenon-131: this compound is unique due to its stable nature and specific nuclear properties, such as its nuclear spin of 3/2, which makes it particularly useful in NMR spectroscopy . Unlike xenon-133, which is radioactive, this compound does not pose radiation hazards, making it safer for certain applications.
Biological Activity
Xenon-131 (Xe-131) is a noble gas that has garnered attention for its unique biological properties, particularly in the fields of anesthesia and neuroprotection. This article explores the biological activity of Xe-131, focusing on its interactions at the molecular level, its anesthetic effects, and its potential therapeutic applications.
Overview of this compound
This compound is a stable isotope of xenon with a nuclear spin of 3/2. It is one of the isotopes used in various medical applications, including as a contrast agent in imaging and in the treatment of certain thyroid diseases. Its biological activity is primarily attributed to its ability to interact with proteins and receptors in the central nervous system.
NMDA Receptor Interaction
One of the primary mechanisms through which Xe-131 exerts its biological effects is via its interaction with the N-methyl-D-aspartate (NMDA) receptor. Research indicates that Xe-131 acts as a competitive antagonist at the glycine binding site of the NMDA receptor. This interaction inhibits excitatory neurotransmission, which is crucial for its anesthetic properties:
- Competitive Binding : Xe-131 competes with glycine for binding at the NMDA receptor, reducing excitatory signaling in neurons .
- Neuroprotective Effects : By inhibiting NMDA receptor activity, Xe-131 reduces excitotoxicity and neuronal injury during hypoxic conditions .
Anesthetic Properties
Xe-131 is recognized for its anesthetic properties due to its low solubility in tissues, allowing for rapid induction and recovery from anesthesia. Studies have shown that Xe-131 can achieve maximum arterial blood concentrations during inhalation anesthesia, with a biphasic elimination profile characterized by rapid initial clearance followed by slower elimination phases .
Study on Xenon-Induced Anesthesia
A study involving seven patients undergoing routine Xe-based general anesthesia reported maximum arterial blood concentrations of 1.3 mmol/L. The elimination kinetics were analyzed using linear regression, revealing a half-life of approximately 2.7 hours post-exposure, indicating that Xe-131 remains traceable for 24 to 48 hours after administration .
Neuroprotective Effects Against OGD Injury
Another significant study focused on the neuroprotective effects of Xe-131 during oxygen-glucose deprivation (OGD). In vitro experiments demonstrated that Xe-131 significantly reduced lactate dehydrogenase release—a marker of cell injury—indicating its protective role against neuronal damage when administered alongside OGD agents .
Data Tables
Parameter | Value |
---|---|
Maximum Blood Concentration | 1.3 mmol/L |
Half-Life | 2.7 hours |
Traceability Duration | 24 to 48 hours |
LDH Release Reduction | Significant at subanesthetic doses |
Proteomic Insights
Recent computational studies have identified numerous proteins that interact with xenon, enhancing our understanding of xenon's biological roles. A large-scale analysis identified 89 unique xenon-interacting proteins, many involved in ATP-driven processes, suggesting that xenon may influence metabolic pathways critical for cellular function .
Q & A
Basic Research Questions
Q. What are the nuclear decay pathways of Xenon-131, and how do they influence its stability in experimental systems?
this compound undergoes beta decay, emitting a beta particle (electron or positron) and an antineutrino, resulting in the formation of iodine-131 (I-131). Its metastable state, Xenon-131m (11.8-day half-life), decays predominantly via internal conversion electrons (98%) and gamma-ray emission (2%). The stability of this compound in experiments depends on its decay constant (λ = ln(2)/t₁/₂) and environmental factors like temperature and radiation shielding. Researchers must account for these decay pathways when designing storage protocols or tracking isotopic purity over time .
Q. How is this compound utilized in medical imaging, and what methodological considerations are critical for its application?
this compound itself is not directly used in imaging, but its decay product, I-131, is employed in thyroid scans and cancer therapy. Researchers must monitor the decay kinetics (e.g., using gamma spectroscopy) to ensure precise dosing and timing for imaging. Key steps include:
- Calibrating detectors for I-131’s 364 keV gamma photons.
- Accounting for biological half-life variations in patient studies.
- Implementing radiation safety protocols due to beta-particle emissions .
Advanced Research Questions
Q. How can researchers design experiments to quantify this compound’s decay kinetics in heterogeneous matrices (e.g., biological tissues or environmental samples)?
Methodology :
- Sample Preparation : Spike matrices with known concentrations of this compound and track decay products (I-131) using liquid scintillation counting or gamma spectrometry.
- Data Collection : Measure activity at regular intervals over 3–4 half-lives (≈35–47 days).
- Analysis : Apply the first-order integrated rate law, , where . Validate linearity via a plot of vs. time; deviations may indicate matrix interference .
Q. What experimental strategies address discrepancies in reported half-life values for Xenon-131m?
While most studies report a half-life of 11.8 days for Xenon-131m, inconsistencies may arise from impurities or detector calibration errors. To resolve this:
- Purification : Use gas chromatography or thermal diffusion to isolate Xenon-131m from isotopic mixtures (e.g., fission xenon) .
- Calibration : Cross-validate decay measurements with reference standards (e.g., NIST-traceable sources).
- Statistical Analysis : Perform replicate experiments (n ≥ 5) and apply error propagation models to refine uncertainty margins .
Q. How can isotopic mixtures containing this compound be purified for nuclear magnetic resonance (NMR) studies, and what challenges arise?
Purification Protocol :
- Gas Separation : Employ cryogenic distillation or laser isotope separation to enrich this compound from natural abundance (21.23%).
- Quality Control : Verify purity via mass spectrometry and monitor for isotopic shifts in NMR spectra (e.g., ¹³¹Xe nuclear spin = 3/2, magnetic moment = 0.692 μ). Challenges :
- Signal broadening due to quadrupole interactions (−0.12 barn quadrupole moment).
- Competition with spin-polarized ¹²⁹Xe in hyperpolarized MRI applications .
Q. Data Contradiction and Analysis
Q. How should researchers reconcile conflicting data on this compound’s beta-decay branching ratios?
Discrepancies in beta/gamma emission ratios often stem from detector efficiency variations or background radiation. To mitigate:
- Use high-purity germanium (HPGe) detectors with energy resolution <2 keV at 1.33 MeV.
- Normalize counts to reference spectra (e.g., ¹³⁷Cs for gamma efficiency curves).
- Apply Monte Carlo simulations (e.g., Geant4) to model detector response and correct for geometric attenuation .
Q. What statistical frameworks are optimal for analyzing time-series decay data of this compound in environmental monitoring?
- Model Selection : Use Akaike Information Criterion (AIC) to compare first-order vs. multi-exponential decay models.
- Uncertainty Quantification : Bootstrap resampling to estimate confidence intervals for half-life measurements.
- Outlier Detection : Apply Grubbs’ test to identify anomalous data points caused by sporadic contamination .
Q. Experimental Design and Reproducibility
Q. What steps ensure reproducibility in synthesizing and characterizing this compound compounds for catalytic studies?
- Synthesis : Document inert-atmosphere techniques (e.g., Schlenk line) to prevent oxidation.
- Characterization : Include X-ray crystallography, NMR (¹²⁹Xe chemical shifts), and isotopic mass spectrometry.
- Reporting : Adhere to the Beilstein Journal’s guidelines:
- Limit main text to 5 critical compounds; archive others in supplementary data.
- Provide crystallographic data (CCDC numbers) and purity metrics (e.g., HPLC traces) .
Q. How can researchers optimize detection limits for this compound in trace-level environmental samples?
- Preconcentration : Use activated charcoal traps or gas-sorption materials (e.g., metal-organic frameworks).
- Analytical Techniques : Pair gas chromatography with mass spectrometry (GC-MS) in selected ion monitoring (SIM) mode.
- Blank Correction : Subtract background signals from ambient xenon (≈0.1 ppm in air) using control samples .
Q. Cross-Disciplinary Applications
Q. What role does this compound play in nuclear forensics, and how is its isotopic signature analyzed?
this compound is a fission product of ²³⁵U and ²³⁹Pu, making it a marker for reactor fuel reprocessing. Methodological steps include:
- Sample Collection : Use high-volume air samplers with xenon-specific adsorbents.
- Isotopic Ratio Analysis : Measure ¹³¹Xe/¹³⁴Xe ratios via accelerator mass spectrometry (AMS) to distinguish natural vs. anthropogenic sources.
- Data Interpretation : Compare ratios to nuclear reactor burn-up codes (e.g., ORIGEN) for attribution .
Properties
IUPAC Name |
xenon-131 | |
---|---|---|
Source | PubChem | |
URL | https://pubchem.ncbi.nlm.nih.gov | |
Description | Data deposited in or computed by PubChem | |
InChI |
InChI=1S/Xe/i1+0 | |
Source | PubChem | |
URL | https://pubchem.ncbi.nlm.nih.gov | |
Description | Data deposited in or computed by PubChem | |
InChI Key |
FHNFHKCVQCLJFQ-IGMARMGPSA-N | |
Source | PubChem | |
URL | https://pubchem.ncbi.nlm.nih.gov | |
Description | Data deposited in or computed by PubChem | |
Canonical SMILES |
[Xe] | |
Source | PubChem | |
URL | https://pubchem.ncbi.nlm.nih.gov | |
Description | Data deposited in or computed by PubChem | |
Isomeric SMILES |
[131Xe] | |
Source | PubChem | |
URL | https://pubchem.ncbi.nlm.nih.gov | |
Description | Data deposited in or computed by PubChem | |
Molecular Formula |
Xe | |
Source | PubChem | |
URL | https://pubchem.ncbi.nlm.nih.gov | |
Description | Data deposited in or computed by PubChem | |
DSSTOX Substance ID |
DTXSID20932930 | |
Record name | (~131~Xe)Xenon | |
Source | EPA DSSTox | |
URL | https://comptox.epa.gov/dashboard/DTXSID20932930 | |
Description | DSSTox provides a high quality public chemistry resource for supporting improved predictive toxicology. | |
Molecular Weight |
130.90508413 g/mol | |
Source | PubChem | |
URL | https://pubchem.ncbi.nlm.nih.gov | |
Description | Data deposited in or computed by PubChem | |
CAS No. |
14683-11-5 | |
Record name | Xenon Xe-131 | |
Source | ChemIDplus | |
URL | https://pubchem.ncbi.nlm.nih.gov/substance/?source=chemidplus&sourceid=0014683115 | |
Description | ChemIDplus is a free, web search system that provides access to the structure and nomenclature authority files used for the identification of chemical substances cited in National Library of Medicine (NLM) databases, including the TOXNET system. | |
Record name | (~131~Xe)Xenon | |
Source | EPA DSSTox | |
URL | https://comptox.epa.gov/dashboard/DTXSID20932930 | |
Description | DSSTox provides a high quality public chemistry resource for supporting improved predictive toxicology. | |
Record name | XENON XE-131 | |
Source | FDA Global Substance Registration System (GSRS) | |
URL | https://gsrs.ncats.nih.gov/ginas/app/beta/substances/EYH1YE0AQ6 | |
Description | The FDA Global Substance Registration System (GSRS) enables the efficient and accurate exchange of information on what substances are in regulated products. Instead of relying on names, which vary across regulatory domains, countries, and regions, the GSRS knowledge base makes it possible for substances to be defined by standardized, scientific descriptions. | |
Explanation | Unless otherwise noted, the contents of the FDA website (www.fda.gov), both text and graphics, are not copyrighted. They are in the public domain and may be republished, reprinted and otherwise used freely by anyone without the need to obtain permission from FDA. Credit to the U.S. Food and Drug Administration as the source is appreciated but not required. | |
Disclaimer and Information on In-Vitro Research Products
Please be aware that all articles and product information presented on BenchChem are intended solely for informational purposes. The products available for purchase on BenchChem are specifically designed for in-vitro studies, which are conducted outside of living organisms. In-vitro studies, derived from the Latin term "in glass," involve experiments performed in controlled laboratory settings using cells or tissues. It is important to note that these products are not categorized as medicines or drugs, and they have not received approval from the FDA for the prevention, treatment, or cure of any medical condition, ailment, or disease. We must emphasize that any form of bodily introduction of these products into humans or animals is strictly prohibited by law. It is essential to adhere to these guidelines to ensure compliance with legal and ethical standards in research and experimentation.