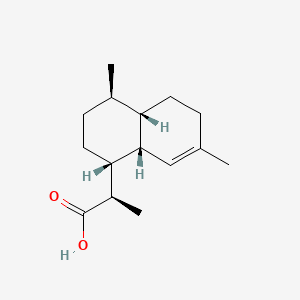
Dihydroartemisinic acid
Overview
Description
Dihydroartemisinic acid is a naturally occurring compound derived from the plant Artemisia annua, commonly known as sweet wormwood. It is a key intermediate in the biosynthesis of artemisinin, a potent antimalarial agent. This compound has garnered significant attention due to its role in the production of artemisinin and its derivatives, which are crucial in the treatment of malaria.
Biochemical Analysis
Biochemical Properties
Dihydroartemisinic acid is involved in several biochemical reactions, primarily as a precursor in the biosynthesis of artemisinin. It interacts with various enzymes, including cytochrome P450 monooxygenase (CYP71AV1) and artemisinic aldehyde dehydrogenase (ALDH1). CYP71AV1 catalyzes the oxidation of amorpha-4,11-diene to artemisinic alcohol, which is further oxidized to artemisinic aldehyde. ALDH1 then converts artemisinic aldehyde to this compound. These interactions are crucial for the production of artemisinin, highlighting the importance of this compound in this biochemical pathway .
Cellular Effects
This compound influences various cellular processes, including cell signaling pathways, gene expression, and cellular metabolism. It has been shown to inhibit the hyphal development of Candida albicans, reducing its damage to oral epithelial cells. This inhibition is mediated through the Ras1-cAMP-Efg1 pathway, which affects energy metabolism and other cellular functions . Additionally, this compound’s role in artemisinin biosynthesis impacts the production of reactive oxygen species (ROS), which can influence cellular metabolism and gene expression .
Molecular Mechanism
The molecular mechanism of this compound involves its conversion to artemisinin through a series of enzymatic and non-enzymatic reactions. The initial oxidation of amorpha-4,11-diene by CYP71AV1 is the rate-limiting step in this pathway. Subsequent steps involve the reduction of artemisinic acid to this compound by ALDH1. The final conversion to artemisinin involves the formation of an endoperoxide bridge, which is crucial for its antimalarial activity. This process may occur through both enzymatic and non-enzymatic pathways, with light and oxygen playing significant roles .
Temporal Effects in Laboratory Settings
In laboratory settings, the stability and degradation of this compound have been studied to understand its long-term effects on cellular function. The compound’s stability is influenced by factors such as temperature, pH, and light exposure. Studies have shown that this compound can be extracted from Artemisia annua using ultrasound-assisted extraction methods, which optimize yield and purity . Over time, this compound can degrade into other compounds, affecting its efficacy and stability in laboratory experiments .
Dosage Effects in Animal Models
The effects of this compound vary with different dosages in animal models. Studies have shown that at lower doses, the compound exhibits antimalarial and anticancer properties, while higher doses may lead to toxic or adverse effects. The threshold for these effects depends on the specific animal model and the duration of exposure. Understanding the dosage effects of this compound is crucial for its potential therapeutic applications .
Metabolic Pathways
This compound is involved in the metabolic pathways leading to the production of artemisinin. The key enzymes in this pathway include CYP71AV1 and ALDH1, which catalyze the conversion of amorpha-4,11-diene to this compound. This pathway also involves the reduction of artemisinic acid to this compound, followed by the formation of artemisinin through a series of oxidation and cyclization reactions .
Transport and Distribution
The transport and distribution of this compound within cells and tissues involve various transporters and binding proteins. In Artemisia annua, lipid transfer proteins (LTPs) play a significant role in the secretion and distribution of sesquiterpene lactones, including this compound. These proteins facilitate the movement of this compound across cell membranes and its accumulation in specific cellular compartments .
Subcellular Localization
This compound is localized in specific subcellular compartments, which can affect its activity and function. In Artemisia annua, the compound is primarily found in glandular trichomes, where it is synthesized and stored. The localization of this compound in these structures is facilitated by lipid transfer proteins and other transport mechanisms. This subcellular localization is crucial for the efficient production and storage of artemisinin precursors .
Preparation Methods
Synthetic Routes and Reaction Conditions: Dihydroartemisinic acid can be synthesized through various methods, including chemical synthesis and biotransformation. One notable method involves the nickel-catalyzed asymmetric hydrogenation of α-substituted acrylic acids, which yields this compound with high enantiomeric excess and diastereomeric ratio . Another approach utilizes whole-cell biotransformation, where enzymes from the this compound pathway are overexpressed in Saccharomyces cerevisiae, converting amorpha-4,11-diene to this compound .
Industrial Production Methods: Industrial production of this compound often involves the use of genetically engineered microorganisms, such as Escherichia coli and Saccharomyces cerevisiae. These microorganisms are engineered to express the necessary enzymes for the biosynthesis of this compound from simpler precursors .
Chemical Reactions Analysis
Types of Reactions: Dihydroartemisinic acid undergoes various chemical reactions, including oxidation, reduction, and photooxygenation. One of the key reactions is the photooxygenation of this compound to form an intermediate hydroperoxide, which is a crucial step in the synthesis of artemisinin .
Common Reagents and Conditions:
Oxidation: Cytochrome P450 enzymes are commonly used for the oxidation of this compound.
Photooxygenation: This reaction requires efficient irradiation and sufficient oxygen supply, often conducted in a two-phase photo-flow reactor.
Major Products: The major product formed from the oxidation of this compound is artemisinin, a highly effective antimalarial agent .
Scientific Research Applications
Dihydroartemisinic acid has a wide range of scientific research applications:
Mechanism of Action
The mechanism of action of dihydroartemisinic acid primarily involves its conversion to artemisinin. Artemisinin exerts its antimalarial effects through the generation of reactive oxygen species (ROS) that damage the cellular components of the malaria parasite. The endoperoxide bridge in artemisinin is responsible for its activity, interacting with iron in the parasite to produce ROS .
Comparison with Similar Compounds
Dihydroartemisinic acid is similar to other artemisinin precursors, such as artemisinic acid and artemisinic alcohol. it is unique in its higher efficiency in producing artemisinin through biotransformation processes . Other similar compounds include:
Artemisinic acid: Another precursor in the artemisinin biosynthesis pathway.
Artemisinic alcohol: A related compound that can also be converted to artemisinin.
This compound stands out due to its higher yield and efficiency in the production of artemisinin, making it a preferred intermediate in industrial applications .
Properties
IUPAC Name |
(2R)-2-[(1R,4R,4aS,8aS)-4,7-dimethyl-1,2,3,4,4a,5,6,8a-octahydronaphthalen-1-yl]propanoic acid | |
---|---|---|
Source | PubChem | |
URL | https://pubchem.ncbi.nlm.nih.gov | |
Description | Data deposited in or computed by PubChem | |
InChI |
InChI=1S/C15H24O2/c1-9-4-6-12-10(2)5-7-13(14(12)8-9)11(3)15(16)17/h8,10-14H,4-7H2,1-3H3,(H,16,17)/t10-,11-,12+,13+,14+/m1/s1 | |
Source | PubChem | |
URL | https://pubchem.ncbi.nlm.nih.gov | |
Description | Data deposited in or computed by PubChem | |
InChI Key |
JYGAZEJXUVDYHI-DGTMBMJNSA-N | |
Source | PubChem | |
URL | https://pubchem.ncbi.nlm.nih.gov | |
Description | Data deposited in or computed by PubChem | |
Canonical SMILES |
CC1CCC(C2C1CCC(=C2)C)C(C)C(=O)O | |
Source | PubChem | |
URL | https://pubchem.ncbi.nlm.nih.gov | |
Description | Data deposited in or computed by PubChem | |
Isomeric SMILES |
C[C@@H]1CC[C@H]([C@@H]2[C@H]1CCC(=C2)C)[C@@H](C)C(=O)O | |
Source | PubChem | |
URL | https://pubchem.ncbi.nlm.nih.gov | |
Description | Data deposited in or computed by PubChem | |
Molecular Formula |
C15H24O2 | |
Source | PubChem | |
URL | https://pubchem.ncbi.nlm.nih.gov | |
Description | Data deposited in or computed by PubChem | |
Molecular Weight |
236.35 g/mol | |
Source | PubChem | |
URL | https://pubchem.ncbi.nlm.nih.gov | |
Description | Data deposited in or computed by PubChem | |
CAS No. |
85031-59-0 | |
Record name | (2R)-2-[(1R,4R,4aS,8aS)-4,7-dimethyl-1,2,3,4,4a,5,6,8a-octahydronaphthalen-1-yl]propanoic acid | |
Source | European Chemicals Agency (ECHA) | |
URL | https://echa.europa.eu/information-on-chemicals | |
Description | The European Chemicals Agency (ECHA) is an agency of the European Union which is the driving force among regulatory authorities in implementing the EU's groundbreaking chemicals legislation for the benefit of human health and the environment as well as for innovation and competitiveness. | |
Explanation | Use of the information, documents and data from the ECHA website is subject to the terms and conditions of this Legal Notice, and subject to other binding limitations provided for under applicable law, the information, documents and data made available on the ECHA website may be reproduced, distributed and/or used, totally or in part, for non-commercial purposes provided that ECHA is acknowledged as the source: "Source: European Chemicals Agency, http://echa.europa.eu/". Such acknowledgement must be included in each copy of the material. ECHA permits and encourages organisations and individuals to create links to the ECHA website under the following cumulative conditions: Links can only be made to webpages that provide a link to the Legal Notice page. | |
Retrosynthesis Analysis
AI-Powered Synthesis Planning: Our tool employs the Template_relevance Pistachio, Template_relevance Bkms_metabolic, Template_relevance Pistachio_ringbreaker, Template_relevance Reaxys, Template_relevance Reaxys_biocatalysis model, leveraging a vast database of chemical reactions to predict feasible synthetic routes.
One-Step Synthesis Focus: Specifically designed for one-step synthesis, it provides concise and direct routes for your target compounds, streamlining the synthesis process.
Accurate Predictions: Utilizing the extensive PISTACHIO, BKMS_METABOLIC, PISTACHIO_RINGBREAKER, REAXYS, REAXYS_BIOCATALYSIS database, our tool offers high-accuracy predictions, reflecting the latest in chemical research and data.
Strategy Settings
Precursor scoring | Relevance Heuristic |
---|---|
Min. plausibility | 0.01 |
Model | Template_relevance |
Template Set | Pistachio/Bkms_metabolic/Pistachio_ringbreaker/Reaxys/Reaxys_biocatalysis |
Top-N result to add to graph | 6 |
Feasible Synthetic Routes
Disclaimer and Information on In-Vitro Research Products
Please be aware that all articles and product information presented on BenchChem are intended solely for informational purposes. The products available for purchase on BenchChem are specifically designed for in-vitro studies, which are conducted outside of living organisms. In-vitro studies, derived from the Latin term "in glass," involve experiments performed in controlled laboratory settings using cells or tissues. It is important to note that these products are not categorized as medicines or drugs, and they have not received approval from the FDA for the prevention, treatment, or cure of any medical condition, ailment, or disease. We must emphasize that any form of bodily introduction of these products into humans or animals is strictly prohibited by law. It is essential to adhere to these guidelines to ensure compliance with legal and ethical standards in research and experimentation.