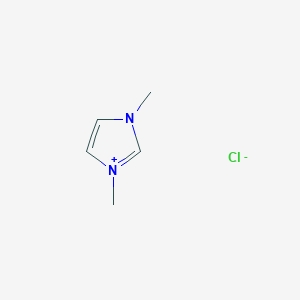
1,3-Dimethylimidazolium Chloride
Overview
Description
1,3-Dimethylimidazolium Chloride is an ionic liquid that has garnered significant interest in various scientific fields due to its unique properties. It is a colorless or slightly yellow crystalline compound that is highly soluble in water and organic solvents. This compound is known for its ability to act as a catalyst in organic synthesis and as a solvent in various chemical reactions.
Mechanism of Action
Target of Action
1,3-Dimethylimidazolium Chloride, also known as [MMIM]Cl, is an example of an ionic liquid . It is used as a solvent and a catalyst for alkylation, esterification, and polymerization reactions . It is also used in the synthesis of various organic compounds .
Mode of Action
The mode of action of this compound is primarily through its interaction with other molecules in the system. It is studied using ab initio methods such as second order Møller–Plesset perturbation theory (MP2), density functional theory (DFT), and classical force field approaches . These methods allow for the investigation of the compound’s properties on different scales .
Biochemical Pathways
It is known that this compound can act as a novel absorbent species with the absorption cycle working fluid . This suggests that it may interact with various biochemical pathways to facilitate the absorption process.
Pharmacokinetics
It is known that this compound is soluble in water , which could potentially influence its bioavailability and pharmacokinetic properties.
Result of Action
It is known that this compound can act as an activating agent in the total synthesis of macroviracin a, cycloviracin b1, and cyclic silanes . This suggests that it may have significant effects at the molecular and cellular levels.
Action Environment
The action of this compound can be influenced by various environmental factors. For instance, it has been proposed that this compound has the potential to be used as a novel absorbent species with the absorption cycle working fluid . This suggests that the compound’s action, efficacy, and stability could be influenced by factors such as the presence of other chemicals, temperature, and pressure.
Biochemical Analysis
Biochemical Properties
1,3-Dimethylimidazolium Chloride plays a crucial role in biochemical reactions, particularly as a solvent and catalyst. It interacts with various enzymes, proteins, and other biomolecules, facilitating or inhibiting biochemical processes. For instance, this compound has been shown to interact with enzymes such as lipases and proteases, enhancing their catalytic activity in non-aqueous media . The nature of these interactions often involves hydrogen bonding and electrostatic interactions, which stabilize the enzyme structure and increase its activity.
Cellular Effects
The effects of this compound on cellular processes are diverse and depend on the concentration and exposure time. At low concentrations, this compound can enhance cell proliferation and metabolic activity by modulating cell signaling pathways and gene expression . At higher concentrations, it may induce cytotoxic effects, leading to cell apoptosis or necrosis. The compound influences cellular metabolism by altering the activity of key metabolic enzymes and affecting the production of reactive oxygen species (ROS).
Molecular Mechanism
The molecular mechanism of action of this compound involves its interaction with cellular membranes and intracellular targets. The compound can penetrate cell membranes, leading to changes in membrane fluidity and permeability . Inside the cell, this compound can bind to specific proteins and enzymes, either inhibiting or activating their function. For example, it has been shown to inhibit acetylcholinesterase, an enzyme involved in neurotransmission, by binding to its active site and preventing substrate access . Additionally, this compound can modulate gene expression by interacting with transcription factors and other regulatory proteins.
Temporal Effects in Laboratory Settings
In laboratory settings, the effects of this compound can change over time due to its stability and degradation. The compound is generally stable under standard laboratory conditions, but prolonged exposure to high temperatures or reactive chemicals can lead to its degradation . Over time, the degradation products of this compound may accumulate and exert different biological effects compared to the parent compound. Long-term studies have shown that continuous exposure to this compound can lead to adaptive cellular responses, such as upregulation of detoxifying enzymes and stress response proteins .
Dosage Effects in Animal Models
The effects of this compound in animal models vary with dosage. At low doses, the compound may have minimal or beneficial effects, such as enhanced metabolic activity and improved organ function . At higher doses, this compound can cause toxic effects, including liver and kidney damage, oxidative stress, and inflammation . Threshold effects have been observed, where a specific dose range triggers a significant biological response, highlighting the importance of dose optimization in experimental studies.
Metabolic Pathways
This compound is involved in various metabolic pathways, primarily through its interaction with metabolic enzymes and cofactors. The compound can affect metabolic flux by altering the activity of key enzymes involved in glycolysis, the tricarboxylic acid cycle, and oxidative phosphorylation . Additionally, this compound can influence metabolite levels by modulating the expression of genes encoding metabolic enzymes and transporters .
Transport and Distribution
The transport and distribution of this compound within cells and tissues are mediated by specific transporters and binding proteins. The compound can be actively transported across cell membranes by membrane transporters, such as organic cation transporters (OCTs) and multidrug resistance proteins (MRPs) . Once inside the cell, this compound can bind to intracellular proteins, affecting its localization and accumulation in specific cellular compartments .
Subcellular Localization
The subcellular localization of this compound is influenced by its chemical properties and interactions with cellular components. The compound can localize to various organelles, including the mitochondria, endoplasmic reticulum, and nucleus . Targeting signals and post-translational modifications may direct this compound to specific subcellular compartments, where it can exert its biological effects. For example, the compound’s interaction with mitochondrial proteins can affect mitochondrial function and energy production .
Preparation Methods
Synthetic Routes and Reaction Conditions
1,3-Dimethylimidazolium Chloride can be synthesized through a gas-liquid phase reaction. The process involves the reaction of 1-methylimidazole with methyl chloride under high-pressure conditions. The reaction is typically carried out in a high-pressure reaction kettle at a temperature of 348.15 K and a pressure of 0.7 MPa .
Industrial Production Methods
In industrial settings, the production of this compound involves similar synthetic routes but on a larger scale. The reaction conditions are optimized to ensure high yield and purity of the product. The compound is then purified through crystallization and filtration processes to obtain the final product .
Chemical Reactions Analysis
Types of Reactions
1,3-Dimethylimidazolium Chloride undergoes various types of chemical reactions, including:
Substitution Reactions: It can participate in nucleophilic substitution reactions where the chloride ion is replaced by other nucleophiles.
Acid-Base Reactions: It can react with bases to form salts.
Complexation Reactions: It can form complexes with metal ions, which are useful in catalysis.
Common Reagents and Conditions
Nucleophiles: Common nucleophiles used in substitution reactions include hydroxide ions, alkoxide ions, and amines.
Bases: Strong bases like sodium hydroxide and potassium hydroxide are used in acid-base reactions.
Metal Ions: Transition metal ions such as palladium and platinum are used in complexation reactions.
Major Products
Substitution Products: Depending on the nucleophile used, various substituted imidazolium compounds can be formed.
Salts: Acid-base reactions result in the formation of imidazolium salts.
Metal Complexes: Complexation reactions yield metal-imidazolium complexes.
Scientific Research Applications
1,3-Dimethylimidazolium Chloride has a wide range of applications in scientific research:
Chemistry: It is used as a catalyst in organic synthesis, particularly in reactions such as alkylation, acylation, and polymerization.
Biology: It is used in the extraction and purification of biomolecules due to its ability to dissolve a wide range of substances.
Medicine: It is being explored for its potential use in drug delivery systems and as a stabilizing agent for pharmaceuticals.
Industry: It is used as a solvent in various industrial processes, including the production of polymers and the extraction of metals
Comparison with Similar Compounds
Similar Compounds
1-Butyl-2,3-Dimethylimidazolium Chloride: Similar in structure but with a butyl group instead of a methyl group.
2-Chloro-1,3-Dimethylimidazolinium Chloride: Known for its high reactivity and used as a precursor in the synthesis of complex organic molecules.
2-Azido-1,3-Dimethylimidazolinium Chloride: Used in diazo-transfer reactions and as a stable reagent in organic synthesis.
Uniqueness
1,3-Dimethylimidazolium Chloride is unique due to its high solubility in both water and organic solvents, making it versatile for various applications. Its ability to form stable complexes with metal ions and act as a catalyst in numerous reactions sets it apart from other similar compounds .
Properties
IUPAC Name |
1,3-dimethylimidazol-1-ium;chloride | |
---|---|---|
Source | PubChem | |
URL | https://pubchem.ncbi.nlm.nih.gov | |
Description | Data deposited in or computed by PubChem | |
InChI |
InChI=1S/C5H9N2.ClH/c1-6-3-4-7(2)5-6;/h3-5H,1-2H3;1H/q+1;/p-1 | |
Source | PubChem | |
URL | https://pubchem.ncbi.nlm.nih.gov | |
Description | Data deposited in or computed by PubChem | |
InChI Key |
IIJSFQFJZAEKHB-UHFFFAOYSA-M | |
Source | PubChem | |
URL | https://pubchem.ncbi.nlm.nih.gov | |
Description | Data deposited in or computed by PubChem | |
Canonical SMILES |
CN1C=C[N+](=C1)C.[Cl-] | |
Source | PubChem | |
URL | https://pubchem.ncbi.nlm.nih.gov | |
Description | Data deposited in or computed by PubChem | |
Molecular Formula |
C5H9ClN2 | |
Source | PubChem | |
URL | https://pubchem.ncbi.nlm.nih.gov | |
Description | Data deposited in or computed by PubChem | |
DSSTOX Substance ID |
DTXSID10453720 | |
Record name | 1,3-Dimethylimidazolium Chloride | |
Source | EPA DSSTox | |
URL | https://comptox.epa.gov/dashboard/DTXSID10453720 | |
Description | DSSTox provides a high quality public chemistry resource for supporting improved predictive toxicology. | |
Molecular Weight |
132.59 g/mol | |
Source | PubChem | |
URL | https://pubchem.ncbi.nlm.nih.gov | |
Description | Data deposited in or computed by PubChem | |
CAS No. |
79917-88-7 | |
Record name | 1,3-Dimethylimidazolium Chloride | |
Source | EPA DSSTox | |
URL | https://comptox.epa.gov/dashboard/DTXSID10453720 | |
Description | DSSTox provides a high quality public chemistry resource for supporting improved predictive toxicology. | |
Record name | 1,3-Dimethylimidazolium Chloride | |
Source | European Chemicals Agency (ECHA) | |
URL | https://echa.europa.eu/information-on-chemicals | |
Description | The European Chemicals Agency (ECHA) is an agency of the European Union which is the driving force among regulatory authorities in implementing the EU's groundbreaking chemicals legislation for the benefit of human health and the environment as well as for innovation and competitiveness. | |
Explanation | Use of the information, documents and data from the ECHA website is subject to the terms and conditions of this Legal Notice, and subject to other binding limitations provided for under applicable law, the information, documents and data made available on the ECHA website may be reproduced, distributed and/or used, totally or in part, for non-commercial purposes provided that ECHA is acknowledged as the source: "Source: European Chemicals Agency, http://echa.europa.eu/". Such acknowledgement must be included in each copy of the material. ECHA permits and encourages organisations and individuals to create links to the ECHA website under the following cumulative conditions: Links can only be made to webpages that provide a link to the Legal Notice page. | |
Retrosynthesis Analysis
AI-Powered Synthesis Planning: Our tool employs the Template_relevance Pistachio, Template_relevance Bkms_metabolic, Template_relevance Pistachio_ringbreaker, Template_relevance Reaxys, Template_relevance Reaxys_biocatalysis model, leveraging a vast database of chemical reactions to predict feasible synthetic routes.
One-Step Synthesis Focus: Specifically designed for one-step synthesis, it provides concise and direct routes for your target compounds, streamlining the synthesis process.
Accurate Predictions: Utilizing the extensive PISTACHIO, BKMS_METABOLIC, PISTACHIO_RINGBREAKER, REAXYS, REAXYS_BIOCATALYSIS database, our tool offers high-accuracy predictions, reflecting the latest in chemical research and data.
Strategy Settings
Precursor scoring | Relevance Heuristic |
---|---|
Min. plausibility | 0.01 |
Model | Template_relevance |
Template Set | Pistachio/Bkms_metabolic/Pistachio_ringbreaker/Reaxys/Reaxys_biocatalysis |
Top-N result to add to graph | 6 |
Feasible Synthetic Routes
Q1: How does 1,3-Dimethylimidazolium Chloride interact with water molecules?
A1: Molecular dynamics simulations reveal that water molecules in mixtures with [DMIM][Cl] tend to remain isolated at low water concentrations. As the water concentration increases, a percolating network of water molecules forms alongside isolated molecules and small clusters [].
Q2: What is the nature of the interaction between this compound and glucose?
A2: [DMIM][Cl] effectively dissolves glucose, primarily through strong hydrogen bonding between the chloride anions and the hydroxyl groups of glucose. The cation also plays a role, albeit smaller, interacting with glucose through van der Waals forces [].
Q3: What is the primary driving force for the interaction between this compound and glucose?
A3: The dominant contributor to the glucose-[DMIM][Cl] interaction energy is the favorable hydrogen bonding between the hydroxyl groups of glucose and chloride anions. This electrostatic interaction is stronger than the van der Waals forces observed between glucose and the [DMIM] cation [].
Q4: What is the molecular formula and weight of this compound?
A4: The molecular formula of this compound is C5H11ClN2, and its molecular weight is 142.62 g/mol [].
Q5: What is known about the charge distribution within this compound?
A5: Computational studies suggest that the ionic charge in [DMIM][Cl] is less than unity, indicating charge transfer from the chloride anion to the cation. This delocalization of the chloride charge is attributed to local interactions and contributes to the liquid's reduced ionic character [].
Q6: What are the structural features of the solid phase formed by confined this compound?
A6: When confined between graphite walls, [DMIM][Cl] forms a unique solid phase with a distinct hydrogen bonding network. This structure is characterized by a hexagonal arrangement of anions around each cation, strong π-π stacking interactions between cations, and a significantly higher melting point compared to the bulk crystal [, ].
Q7: How does confinement between graphite walls affect the phase behavior of this compound?
A7: Molecular dynamics simulations show that a monolayer of [DMIM][Cl] confined between graphite walls undergoes a first-order freezing transition at 425 K. This transition, driven by changes in the distance between the walls, results in a solid monolayer with a hydrogen-bonded network structure distinct from the bulk crystal [].
Q8: How does the presence of this compound affect the micellization of sodium dodecyl sulfate (SDS) in aqueous solutions?
A8: Adding [DMIM][Cl] to aqueous SDS solutions influences SDS micellization, making it more thermodynamically favorable. This effect is attributed to stronger interactions between SDS molecules due to the presence of the IL, potentially through incorporation into the micellar structure [].
Q9: Can this compound be used in the synthesis of specific molecules?
A9: Yes, [DMIM][Cl] plays a crucial role in the one-pot synthesis of glycosyl benzoates from unprotected sugars in aqueous solutions. This process, involving thiobenzoic acid and triethylamine, highlights the potential of [DMIM][Cl] in facilitating efficient carbohydrate synthesis [].
Q10: How has Car-Parrinello Molecular Dynamics (CPMD) been used to study this compound?
A10: CPMD simulations have been instrumental in investigating various aspects of [DMIM][Cl], including:
- Local Liquid Structure: Revealing the presence of hydrogen bonding and providing insights into cation-anion and cation-cation distributions [, ].
- Electrostatic Properties: Analyzing molecular polarization, revealing fluctuating dipole moments of ions influenced by the immediate surroundings [].
- Interfacial Behavior: Studying the formation of interfacial layers at the neutral graphite monolayer-[DMIM][Cl] interface, highlighting the enrichment of cations at the surface [].
Q11: What has been learned about this compound from ab initio molecular dynamics simulations?
A11: Ab initio molecular dynamics simulations have provided valuable insights into:
- Solid-Liquid Phase Transition: Predicting changes in atomic structure during phase transition and its effect on the Compton profile, with intermolecular changes being the main contributors [].
- Structural Properties: Examining the radial distribution functions, hydrogen bonding patterns, and charge transfer phenomena within the liquid [].
- Power Spectra: Analyzing the vibrational modes of the liquid and correlating them with hydrogen bonding strength and experimental IR/Raman spectra [].
Q12: How do ionic charges impact the simulation results of this compound?
A12: Simulations using scaled charges for [DMIM][Cl] reveal that assuming ionic charges of +/-1 e can overestimate intermolecular attractions, leading to inaccurate predictions of structure, dynamics, and cohesive energy densities. Employing scaled charges closer to +/- 0.7 e shows better agreement with ab initio data [].
Q13: How is the Adaptive Resolution Simulation (AdResS) method used to study this compound?
A13: The AdResS method, particularly its grand canonical-like version (GC-AdResS), enables open boundary molecular dynamics simulations of [DMIM][Cl] by partitioning the system into an atomistically detailed subsystem and a coarse-grained reservoir, allowing for efficient and accurate simulations [].
Q14: How does the choice of anion affect the properties of 1,3-dimethylimidazolium-based ionic liquids?
A14: The choice of anion significantly influences the properties of [DMIM]-based ILs. For example, [DMIM] hexafluorophosphate exhibits greater hydrophobicity compared to [DMIM] chloride, as evidenced by contrasting behavior in excess volumes and enthalpies of mixing with water [].
Q15: Can this compound be used to mitigate the toxicity of essential oils?
A15: Research shows that using [DMIM][Cl] as a co-solvent during the hydrodistillation of essential oils, such as nutmeg oil, can potentially reduce the extraction of toxic phenylpropanoids while improving the yield of desirable components [].
Q16: What is the impact of pore size and loading on the properties of this compound confined in nanoporous carbon materials?
A16: Molecular dynamics simulations of [DMIM][Cl] confined within nanoporous CMK-3 carbon reveal that pore size and loading significantly impact the IL's structural and dynamic properties. The IL forms distinct layers within the pores, and its density profile along the pore axis becomes heterogeneous at low pore loadings [].
Q17: How do ionic liquids like this compound screen ion-electrode interactions?
A17: [DMIM][Cl] and similar ILs exhibit a complex screening behavior of ion-electrode interactions. Instead of the monotonic decrease predicted by simpler models, the free energy profiles of dissolved charged probes near a charged surface (e.g., graphene) show oscillations, indicating a complex interplay of forces [].
Q18: What is the role of hydrogen bonding in determining the properties of this compound?
A18: Hydrogen bonding plays a crucial role in shaping the structural and dynamic properties of [DMIM][Cl]. The strength and nature of these interactions, often studied through power spectra analysis of ab initio molecular dynamics simulations, directly influence the liquid's behavior [].
Disclaimer and Information on In-Vitro Research Products
Please be aware that all articles and product information presented on BenchChem are intended solely for informational purposes. The products available for purchase on BenchChem are specifically designed for in-vitro studies, which are conducted outside of living organisms. In-vitro studies, derived from the Latin term "in glass," involve experiments performed in controlled laboratory settings using cells or tissues. It is important to note that these products are not categorized as medicines or drugs, and they have not received approval from the FDA for the prevention, treatment, or cure of any medical condition, ailment, or disease. We must emphasize that any form of bodily introduction of these products into humans or animals is strictly prohibited by law. It is essential to adhere to these guidelines to ensure compliance with legal and ethical standards in research and experimentation.