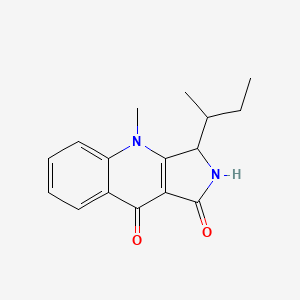
Quinolactacin A
Overview
Description
Quinolactacin A is a novel fungal alkaloid that features a unique quinolone skeleton conjugated with a gamma-lactam ring. It was first isolated from the cultured broth of Penicillium species EPF-6, which was obtained from the larvae of the mulberry pyralid (Margaronia pyloalis Welker). This compound exhibits significant biological activity, including inhibitory effects on tumor necrosis factor production by murine macrophages and macrophage-like J774.1 cells stimulated with lipopolysaccharide .
Scientific Research Applications
Quinolactacin A has several scientific research applications, including:
Chemistry: It serves as a model compound for studying the biosynthesis and chemical reactivity of quinolone and gamma-lactam hybrids.
Biology: this compound is used to investigate the biological pathways involved in tumor necrosis factor production and inhibition.
Medicine: Due to its inhibitory effects on tumor necrosis factor production, this compound has potential therapeutic applications in treating inflammatory diseases and cancer.
Industry: The unique structure of this compound makes it a valuable compound for developing new pharmaceuticals and agrochemicals.
Mechanism of Action
Target of Action
Quinolactacin A is a novel fungal alkaloid that primarily targets acetylcholinesterase and tumor necrosis factor (TNF) . Acetylcholinesterase is an enzyme involved in the termination of impulse transmission at cholinergic synapses, while TNF is a cell signaling protein involved in systemic inflammation and immune response.
Mode of Action
This compound exhibits inhibitory activity against acetylcholinesterase and TNF production . By inhibiting acetylcholinesterase, it prevents the breakdown of acetylcholine, a neurotransmitter, thereby increasing both the level and duration of action of this neurotransmitter. On the other hand, by inhibiting TNF production, it can modulate inflammatory responses, which could be beneficial in conditions like Alzheimer’s disease and cancer .
Biochemical Pathways
The biosynthesis of this compound involves a unique β-keto acid (N-methyl-2-aminobenzoylacetate) precursor that is derived from the primary metabolite L-kynurenine via methylation, oxidative decarboxylation, and amide hydrolysis reactions . This pathway provides a new substrate, β-keto acid, for non-ribosomal peptide synthetases (NRPSs) QulA and QulB .
Result of Action
The result of this compound’s action is the increased level and duration of action of acetylcholine and the modulation of inflammatory responses. This could potentially alleviate symptoms of Alzheimer’s disease and inhibit tumor growth .
Future Directions
Biochemical Analysis
Biochemical Properties
Quinolactacin A plays a crucial role in biochemical reactions, particularly in the inhibition of TNF production. It interacts with various enzymes, proteins, and other biomolecules. One of the primary interactions is with macrophages and macrophage-like cells, where this compound inhibits the production of TNF induced by lipopolysaccharide (LPS) stimulation . This interaction is significant as TNF is a key cytokine involved in inflammation and immune responses. Additionally, this compound has been shown to inhibit acetylcholinesterase, an enzyme responsible for breaking down acetylcholine, thereby affecting neurotransmission .
Cellular Effects
This compound exerts various effects on different cell types and cellular processes. In macrophages and macrophage-like cells, it inhibits TNF production, which plays a critical role in modulating inflammatory responses . This inhibition can impact cell signaling pathways, gene expression, and cellular metabolism. For instance, by reducing TNF levels, this compound can alter the expression of genes involved in inflammation and immune responses. Moreover, its inhibitory effect on acetylcholinesterase can influence neuronal cells by affecting neurotransmitter levels and signaling pathways .
Molecular Mechanism
The molecular mechanism of this compound involves its interaction with specific biomolecules and enzymes. It binds to the active site of acetylcholinesterase, inhibiting its activity and preventing the breakdown of acetylcholine . This inhibition leads to increased levels of acetylcholine, which can enhance cholinergic signaling. Additionally, this compound interacts with macrophages, inhibiting the signaling pathways that lead to TNF production. This inhibition is likely mediated through the suppression of nuclear factor-kappa B (NF-κB) activation, a key transcription factor involved in TNF gene expression .
Temporal Effects in Laboratory Settings
In laboratory settings, the effects of this compound have been observed to change over time. The compound exhibits stability under standard laboratory conditions, but its activity can degrade over extended periods . Long-term studies have shown that this compound maintains its inhibitory effects on TNF production and acetylcholinesterase activity for several weeks. Prolonged exposure to environmental factors such as light and temperature can lead to gradual degradation and reduced efficacy .
Dosage Effects in Animal Models
The effects of this compound vary with different dosages in animal models. At lower doses, it effectively inhibits TNF production without causing significant adverse effects . At higher doses, this compound can exhibit toxic effects, including hepatotoxicity and neurotoxicity . These toxic effects are dose-dependent and highlight the importance of determining the optimal dosage for therapeutic applications. Threshold effects have been observed, where doses above a certain level result in a sharp increase in toxicity .
Metabolic Pathways
This compound is involved in several metabolic pathways, primarily related to its biosynthesis and degradation. The compound is synthesized through the combination of amino acids such as valine and isoleucine, along with anthranilic acid and acetic acid . These precursors are incorporated into the quinolone skeleton and γ-lactam ring structure. This compound interacts with various enzymes during its biosynthesis, including those involved in the incorporation of 13C-labeled precursors . Additionally, its degradation involves enzymatic processes that break down the quinolone and lactam structures .
Transport and Distribution
Within cells and tissues, this compound is transported and distributed through interactions with specific transporters and binding proteins. It is known to accumulate in macrophages and neuronal cells, where it exerts its inhibitory effects . The compound’s distribution is influenced by its lipophilic nature, allowing it to cross cell membranes and reach intracellular targets. Transporters such as organic anion-transporting polypeptides (OATPs) may play a role in its cellular uptake and distribution .
Subcellular Localization
This compound exhibits specific subcellular localization, which is crucial for its activity and function. It is primarily localized in the cytoplasm of macrophages and neuronal cells . This localization is facilitated by targeting signals and post-translational modifications that direct the compound to specific cellular compartments. In macrophages, this compound accumulates in the cytoplasm, where it interacts with signaling pathways involved in TNF production . In neuronal cells, it localizes to regions involved in cholinergic signaling, enhancing its inhibitory effects on acetylcholinesterase .
Preparation Methods
Synthetic Routes and Reaction Conditions: The biosynthesis of Quinolactacin A involves feeding experiments using several carbon-13 single-labeled precursors such as sodium [1-13C]acetate, DL-[1-13C]-isoleucine, L-[methyl-13C]methionine, and sodium [1-13C]-anthranilate, along with D-[U-13C]glucose . The biosynthetic pathway includes the incorporation of these precursors into the quinolone skeleton and gamma-lactam ring through a series of enzymatic reactions.
Industrial Production Methods: Industrial production of this compound is not well-documented, but it is likely to involve large-scale fermentation processes using Penicillium species. Optimization of fermentation conditions, such as nutrient composition, pH, temperature, and aeration, would be essential to maximize the yield of this compound.
Chemical Reactions Analysis
Types of Reactions: Quinolactacin A undergoes various chemical reactions, including:
Oxidation: The quinolone skeleton can be oxidized under specific conditions.
Reduction: The gamma-lactam ring can be reduced to form different derivatives.
Substitution: The quinolone ring can undergo substitution reactions with various nucleophiles.
Common Reagents and Conditions:
Oxidation: Common oxidizing agents such as potassium permanganate or chromium trioxide can be used.
Reduction: Reducing agents like lithium aluminum hydride or sodium borohydride are employed.
Substitution: Nucleophiles such as amines or thiols can be used under basic conditions.
Major Products: The major products formed from these reactions include oxidized quinolactacin derivatives, reduced gamma-lactam derivatives, and substituted quinolone compounds.
Comparison with Similar Compounds
Quinolactacin B: Another quinolone-gamma-lactam hybrid with different substituents.
Quinolactacin C: Similar structure but with variations in the gamma-lactam ring.
Quinolactacin A stands out due to its potent biological activity and potential therapeutic applications.
Properties
IUPAC Name |
3-butan-2-yl-4-methyl-2,3-dihydropyrrolo[3,4-b]quinoline-1,9-dione | |
---|---|---|
Source | PubChem | |
URL | https://pubchem.ncbi.nlm.nih.gov | |
Description | Data deposited in or computed by PubChem | |
InChI |
InChI=1S/C16H18N2O2/c1-4-9(2)13-14-12(16(20)17-13)15(19)10-7-5-6-8-11(10)18(14)3/h5-9,13H,4H2,1-3H3,(H,17,20) | |
Source | PubChem | |
URL | https://pubchem.ncbi.nlm.nih.gov | |
Description | Data deposited in or computed by PubChem | |
InChI Key |
FLHQAMWKNPOTDV-UHFFFAOYSA-N | |
Source | PubChem | |
URL | https://pubchem.ncbi.nlm.nih.gov | |
Description | Data deposited in or computed by PubChem | |
Canonical SMILES |
CCC(C)C1C2=C(C(=O)C3=CC=CC=C3N2C)C(=O)N1 | |
Source | PubChem | |
URL | https://pubchem.ncbi.nlm.nih.gov | |
Description | Data deposited in or computed by PubChem | |
Molecular Formula |
C16H18N2O2 | |
Source | PubChem | |
URL | https://pubchem.ncbi.nlm.nih.gov | |
Description | Data deposited in or computed by PubChem | |
Molecular Weight |
270.33 g/mol | |
Source | PubChem | |
URL | https://pubchem.ncbi.nlm.nih.gov | |
Description | Data deposited in or computed by PubChem | |
Retrosynthesis Analysis
AI-Powered Synthesis Planning: Our tool employs the Template_relevance Pistachio, Template_relevance Bkms_metabolic, Template_relevance Pistachio_ringbreaker, Template_relevance Reaxys, Template_relevance Reaxys_biocatalysis model, leveraging a vast database of chemical reactions to predict feasible synthetic routes.
One-Step Synthesis Focus: Specifically designed for one-step synthesis, it provides concise and direct routes for your target compounds, streamlining the synthesis process.
Accurate Predictions: Utilizing the extensive PISTACHIO, BKMS_METABOLIC, PISTACHIO_RINGBREAKER, REAXYS, REAXYS_BIOCATALYSIS database, our tool offers high-accuracy predictions, reflecting the latest in chemical research and data.
Strategy Settings
Precursor scoring | Relevance Heuristic |
---|---|
Min. plausibility | 0.01 |
Model | Template_relevance |
Template Set | Pistachio/Bkms_metabolic/Pistachio_ringbreaker/Reaxys/Reaxys_biocatalysis |
Top-N result to add to graph | 6 |
Feasible Synthetic Routes
Disclaimer and Information on In-Vitro Research Products
Please be aware that all articles and product information presented on BenchChem are intended solely for informational purposes. The products available for purchase on BenchChem are specifically designed for in-vitro studies, which are conducted outside of living organisms. In-vitro studies, derived from the Latin term "in glass," involve experiments performed in controlled laboratory settings using cells or tissues. It is important to note that these products are not categorized as medicines or drugs, and they have not received approval from the FDA for the prevention, treatment, or cure of any medical condition, ailment, or disease. We must emphasize that any form of bodily introduction of these products into humans or animals is strictly prohibited by law. It is essential to adhere to these guidelines to ensure compliance with legal and ethical standards in research and experimentation.