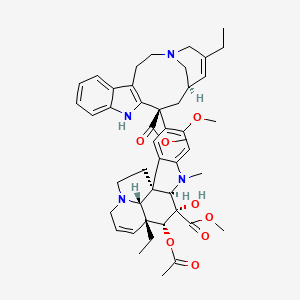
Anhydrovinblastine
Overview
Description
Anhydrovinblastine has been used in trials studying the treatment of Unspecified Adult Solid Tumor, Protocol Specific.
This compound is a semisynthetic derivative of the vinca alkaloid vinblastine, with potential antineoplastic activity. Like vinblastine, this compound targets and binds to tubulin and inhibits microtubule formation, resulting in disruption of mitotic spindle assembly and causing tumor cell cycle arrest in the M phase.
Mechanism of Action
Target of Action
Anhydrovinblastine, also known as Anhydrovincaleukoblastine, is a type of chemotherapy that primarily targets cancer cells . It works by stopping the cancer cells from separating into two new cells, thereby blocking the growth of the cancer .
Mode of Action
It is known that this compound and other vinca alkaloids exert their cytotoxic effects by binding to tubulin, the protein subunit of the microtubules that form the mitotic spindle . This binding inhibits tubulin polymerization into microtubules, which are essential for cell division .
Biochemical Pathways
This compound affects the biochemical pathways involved in cell division. By binding to tubulin, it disrupts the formation of microtubules, structures that are crucial for the separation of chromosomes during cell division . This disruption prevents the cancer cells from dividing and growing . The biosynthesis of this compound involves various enzymes and metabolic pathways in the plant Catharanthus roseus .
Pharmacokinetics
The pharmacokinetics of this compound were characterized by a two-compartment model, with a mean clearance of 26.4 l/h per m² and a median terminal half-life of 18 hours . These properties impact the bioavailability of the drug, determining how quickly it is cleared from the body and how long it remains effective .
Result of Action
The primary result of this compound’s action is the inhibition of cancer cell growth. By preventing the formation of microtubules, it stops the cancer cells from dividing and proliferating . This can lead to the shrinkage of tumors and a reduction in cancer symptoms .
Action Environment
The action of this compound can be influenced by various environmental factors. For instance, the presence of other drugs can affect its efficacy and stability . Additionally, the physiological environment within the body, such as the pH and the presence of certain enzymes, can also impact the action of this compound .
Biochemical Analysis
Biochemical Properties
Anhydrovinblastine plays a crucial role in biochemical reactions, particularly in the biosynthesis of vinblastine. It interacts with various enzymes, proteins, and biomolecules. One of the key enzymes involved is α-3′,4′-anhydrovinblastine synthase (AVLBS), which catalyzes the coupling of vindoline and catharanthine to produce this compound . This enzyme belongs to the class III basic peroxidases and is essential for the formation of the dimeric structure of this compound . Additionally, this compound interacts with other intermediate compounds in the biosynthetic pathway, highlighting its importance in the production of therapeutic alkaloids .
Cellular Effects
This compound exerts significant effects on various types of cells and cellular processes. It influences cell function by interfering with microtubule dynamics, leading to mitotic arrest and cell death . This compound affects cell signaling pathways, gene expression, and cellular metabolism. For instance, this compound has been shown to inhibit the formation of the mitotic spindle, thereby blocking cell division . It also impacts the DNA repair and RNA synthesis mechanisms, further contributing to its cytotoxic effects .
Molecular Mechanism
The molecular mechanism of this compound involves its interaction with tubulin, a key protein in the formation of microtubules . This compound binds to the microtubular proteins of the mitotic spindle, leading to the crystallization of the microtubule and subsequent mitotic arrest or cell death . This interaction inhibits the polymerization of tubulin, preventing the formation of functional microtubules and disrupting the cell cycle . Additionally, this compound may influence gene expression by modulating the activity of transcription factors and other regulatory proteins .
Temporal Effects in Laboratory Settings
In laboratory settings, the effects of this compound change over time. The compound’s stability and degradation are critical factors influencing its long-term effects on cellular function. Studies have shown that this compound remains stable under specific conditions, but its degradation can lead to the formation of inactive metabolites . Long-term exposure to this compound in in vitro and in vivo studies has demonstrated sustained cytotoxic effects, with potential implications for cancer therapy .
Dosage Effects in Animal Models
The effects of this compound vary with different dosages in animal models. At lower doses, this compound exhibits significant antitumor activity without causing severe toxicity . At higher doses, the compound can induce toxic or adverse effects, including neurotoxicity and myelosuppression . Threshold effects have been observed, where the therapeutic benefits of this compound are maximized at specific dosage levels, while minimizing adverse effects .
Metabolic Pathways
This compound is involved in the metabolic pathways of vinca alkaloids. It is synthesized through the coupling of vindoline and catharanthine, catalyzed by α-3′,4′-anhydrovinblastine synthase . This enzyme-mediated reaction is a key step in the biosynthesis of vinblastine and other related alkaloids . The metabolic pathways of this compound also involve various intermediate compounds and cofactors, contributing to the overall production of therapeutic alkaloids .
Transport and Distribution
This compound is transported and distributed within cells and tissues through specific transporters and binding proteins. In Catharanthus roseus, the compound is localized in the vacuoles of leaf cells, where it undergoes further biosynthetic transformations . The transport of this compound involves its movement from the epidermis to laticifers/idioblasts, where it is condensed with vindoline to form the dimeric structure . This transport mechanism ensures the efficient distribution and accumulation of this compound within the plant tissues .
Subcellular Localization
The subcellular localization of this compound is primarily within the vacuoles of Catharanthus roseus cells . The enzyme α-3′,4′-anhydrovinblastine synthase, responsible for its synthesis, is also localized in the vacuoles . This compartmentalization is crucial for the biosynthesis and accumulation of this compound, as it allows for the efficient conversion of precursor molecules into the final dimeric structure . The vacuolar localization also protects the compound from potential degradation and ensures its availability for further biosynthetic processes .
Scientific Research Applications
Chemical Structure and Mechanism of Action
Anhydrovinblastine is characterized by a double bond at the 3',4' position of the caranthine nucleus, distinguishing it from vinblastine, which has a hydroxyl group at the same position. This structural modification contributes to its enhanced antitumor efficacy and reduced toxicity compared to vinblastine and vincristine .
Antineoplastic Properties
AHVB has been extensively studied for its antitumor properties across various cancer types. Key findings include:
- Cytotoxicity : In comparative studies, AHVB demonstrated significant cytotoxic effects against tumor cell lines such as SKOV3 and C-4, with IC50 values of 4.0 µM and 0.02 µM respectively . This indicates a potent ability to inhibit cancer cell proliferation.
- In Vivo Efficacy : In rodent models, AHVB showed improved anti-tumor activity when administered at higher doses than vincristine. For instance, a single intravenous injection of AHVB at 40 mg/kg resulted in better outcomes than the maximum tolerated dose of vincristine (3 mg/kg) in terms of tumor weight reduction and long-term survival rates .
- Specific Cancer Types : Research indicates that AHVB is particularly effective in treating cervical and lung cancers, with studies demonstrating its ability to delay tumor growth significantly compared to traditional vinca alkaloids .
Comparative Efficacy with Other Vinca Alkaloids
A series of studies have compared AHVB with other vinca alkaloids like vincristine and navelbine:
Compound | Maximum Tolerated Dose (mg/kg) | IC50 (SKOV3) | IC50 (C-4) | Observations |
---|---|---|---|---|
This compound | 40 | 4.0 µM | 0.02 µM | Better survival rates in animal models |
Vincristine | 3 | N/A | N/A | Higher toxicity limits treatment options |
Navelbine | ~40 | N/A | N/A | Comparable efficacy but higher toxicity than AHVB |
This table illustrates the relative advantages of AHVB in terms of dosage tolerance and cytotoxicity.
Clinical Implications
The findings surrounding AHVB suggest several clinical implications:
- Combination Therapy : Given its reduced toxicity profile, AHVB may be used in combination with other chemotherapeutic agents to enhance overall efficacy while minimizing adverse effects.
- Targeted Cancer Therapies : The specific activity against certain cancer types positions AHVB as a candidate for targeted therapies, particularly in cases resistant to conventional treatments.
Case Studies and Research Findings
- Rodent Model Studies : In one study, rodents treated with AHVB exhibited a significant reduction in tumor weight compared to those treated with vincristine or saline controls. The long-term survival rate was notably higher among those receiving AHVB .
- Cytotoxicity Assays : Cytotoxicity assays conducted on various tumor cell lines have consistently shown that AHVB is more effective than vincristine and navelbine, supporting its potential as a preferred treatment option in clinical settings .
- Biosynthetic Pathways : Research into the biosynthesis of indole alkaloids has highlighted the role of AHVB as an intermediate compound, further emphasizing its relevance in pharmacognosy and medicinal chemistry .
Q & A
Basic Research Questions
Q. What are the standard methodologies for synthesizing Anhydrovinblastine, and how do reaction conditions influence stereochemical outcomes?
this compound synthesis typically involves electrochemical coupling of catharanthine and vindoline precursors. Key variables include temperature, inert gas environment, and reaction time. For example, the active 16′-S epimer dominates at low temperatures (≤50°C), while higher temperatures favor the inactive 16′-R epimer. Researchers must validate stereochemistry via NMR and X-ray crystallography, as even optimal conditions yield ~10% undesired epimer . Electrochemical methods (e.g., anodic fragmentation) are preferred for scalability but require rigorous pH control to avoid side reactions .
Q. How can researchers validate the purity and identity of this compound derivatives in cytotoxicity assays?
Use a combination of HPLC (≥95% purity threshold), mass spectrometry (exact mass confirmation), and 1H/13C NMR for structural elucidation. For novel derivatives, include elemental analysis and compare spectral data with established analogs (e.g., 5′-nor-anhydrovinblastine). In cytotoxicity studies, pair these with positive controls (e.g., vinblastine) and replicate experiments across cell lines (e.g., human lung adenocarcinoma) to ensure reproducibility .
Q. What are the best practices for designing in vitro studies to assess this compound’s antiproliferative effects?
- Cell lines : Use panels representing diverse cancer types (e.g., leukemia, breast, lung).
- Dose ranges : Start with 0.1–100 μM, based on IC50 values reported for vinblastine analogs.
- Assay duration : 48–72 hours to capture G2/M phase arrest, confirmed via flow cytometry.
- Data normalization : Include vehicle controls and use MTT/WST-1 assays for viability. Document batch-to-batch variability in compound solubility (e.g., DMSO concentration ≤0.1%) .
Advanced Research Questions
Q. How can conflicting data on this compound’s metabolic stability be resolved in preclinical models?
Contradictions often arise from interspecies differences in Phase I/II metabolism. For example, rat models show extensive hydroxylation and glucuronidation, whereas human hepatocytes may prioritize sulfation. To address this:
- Perform comparative LC-MS/MS metabolomic profiling across species.
- Use isotope-labeled this compound to track metabolite formation.
- Apply physiologically based pharmacokinetic (PBPK) modeling to extrapolate human clearance .
Q. What strategies optimize the structure-activity relationship (SAR) of this compound analogs for reduced neurotoxicity?
- Modification sites : Introduce substituents at C21′ (e.g., cyano groups) to enhance tubulin binding selectivity.
- In silico tools : Use molecular docking (e.g., Autodock Vina) to predict interactions with β-tubulin’s vinca domain.
- In vivo validation : Test analogs in rodent models for motor neuron toxicity (e.g., rotarod tests) alongside efficacy studies .
Q. How should researchers design experiments to investigate this compound’s synergy with DNA-damaging agents (e.g., cisplatin)?
- Experimental design : Use factorial ANOVA to assess additive vs. synergistic effects.
- Endpoint selection : Measure apoptosis (Annexin V/PI staining) and DNA repair markers (e.g., γH2AX).
- Mechanistic follow-up : Perform RNA-seq to identify pathways (e.g., p53, MAPK) modulated by combination therapy .
Q. What methodological considerations are critical for reproducible in vivo pharmacokinetic studies of this compound?
- Dosing routes : Intravenous administration avoids first-pass metabolism but requires stability in saline.
- Sampling intervals : Collect plasma at 0.5, 1, 2, 4, 8, 12, and 24 hours post-dose.
- Analytical validation : Ensure LC-MS/MS methods detect this compound and major metabolites (LOQ ≤1 ng/mL) .
Q. Data Analysis & Reproducibility
Q. How can researchers address batch-to-batch variability in this compound’s biological activity?
- Quality control : Implement strict SOPs for synthesis and storage (−80°C under argon).
- Bioactivity normalization : Express IC50 values relative to a reference batch.
- Metadata documentation : Track solvent purity, humidity, and catalyst lots in supplementary materials .
Q. What statistical approaches are recommended for analyzing contradictory cytotoxicity data across independent studies?
- Apply meta-analysis using random-effects models to account for heterogeneity.
- Stratify data by cell type, passage number, and assay methodology.
- Use funnel plots to detect publication bias .
Q. Ethical & Reporting Standards
Q. How should researchers report negative or inconclusive results in this compound studies?
Properties
CAS No. |
38390-45-3 |
---|---|
Molecular Formula |
C46H56N4O8 |
Molecular Weight |
793.0 g/mol |
IUPAC Name |
methyl (1R,10S,12R)-11-acetyloxy-12-ethyl-4-[(13S)-17-ethyl-13-methoxycarbonyl-1,11-diazatetracyclo[13.3.1.04,12.05,10]nonadeca-4(12),5,7,9,16-pentaen-13-yl]-10-hydroxy-5-methoxy-8-methyl-8,16-diazapentacyclo[10.6.1.01,9.02,7.016,19]nonadeca-2,4,6,13-tetraene-10-carboxylate |
InChI |
InChI=1S/C46H56N4O8/c1-8-28-21-29-24-45(41(52)56-6,37-31(15-19-49(25-28)26-29)30-13-10-11-14-34(30)47-37)33-22-32-35(23-36(33)55-5)48(4)39-44(32)17-20-50-18-12-16-43(9-2,38(44)50)40(58-27(3)51)46(39,54)42(53)57-7/h10-14,16,21-23,29,38-40,47,54H,8-9,15,17-20,24-26H2,1-7H3/t29?,38?,39?,40?,43-,44-,45+,46+/m1/s1 |
InChI Key |
FFRFGVHNKJYNOV-YMNHSNPGSA-N |
SMILES |
CCC1=CC2CC(C3=C(CCN(C2)C1)C4=CC=CC=C4N3)(C5=C(C=C6C(=C5)C78CCN9C7C(C=CC9)(C(C(C8N6C)(C(=O)OC)O)OC(=O)C)CC)OC)C(=O)OC |
Isomeric SMILES |
CCC1=CC2C[C@@](C3=C(CCN(C2)C1)C4=CC=CC=C4N3)(C5=C(C=C6C(=C5)[C@]78CCN9C7[C@@](C=CC9)(C([C@@](C8N6C)(C(=O)OC)O)OC(=O)C)CC)OC)C(=O)OC |
Canonical SMILES |
CCC1=CC2CC(C3=C(CCN(C2)C1)C4=CC=CC=C4N3)(C5=C(C=C6C(=C5)C78CCN9C7C(C=CC9)(C(C(C8N6C)(C(=O)OC)O)OC(=O)C)CC)OC)C(=O)OC |
Key on ui other cas no. |
38390-45-3 |
Synonyms |
3',4'-anhydrovinblastine 3',4'-anhydrovinblastine, (18'alpha)-isome |
Origin of Product |
United States |
Retrosynthesis Analysis
AI-Powered Synthesis Planning: Our tool employs the Template_relevance Pistachio, Template_relevance Bkms_metabolic, Template_relevance Pistachio_ringbreaker, Template_relevance Reaxys, Template_relevance Reaxys_biocatalysis model, leveraging a vast database of chemical reactions to predict feasible synthetic routes.
One-Step Synthesis Focus: Specifically designed for one-step synthesis, it provides concise and direct routes for your target compounds, streamlining the synthesis process.
Accurate Predictions: Utilizing the extensive PISTACHIO, BKMS_METABOLIC, PISTACHIO_RINGBREAKER, REAXYS, REAXYS_BIOCATALYSIS database, our tool offers high-accuracy predictions, reflecting the latest in chemical research and data.
Strategy Settings
Precursor scoring | Relevance Heuristic |
---|---|
Min. plausibility | 0.01 |
Model | Template_relevance |
Template Set | Pistachio/Bkms_metabolic/Pistachio_ringbreaker/Reaxys/Reaxys_biocatalysis |
Top-N result to add to graph | 6 |
Feasible Synthetic Routes
Disclaimer and Information on In-Vitro Research Products
Please be aware that all articles and product information presented on BenchChem are intended solely for informational purposes. The products available for purchase on BenchChem are specifically designed for in-vitro studies, which are conducted outside of living organisms. In-vitro studies, derived from the Latin term "in glass," involve experiments performed in controlled laboratory settings using cells or tissues. It is important to note that these products are not categorized as medicines or drugs, and they have not received approval from the FDA for the prevention, treatment, or cure of any medical condition, ailment, or disease. We must emphasize that any form of bodily introduction of these products into humans or animals is strictly prohibited by law. It is essential to adhere to these guidelines to ensure compliance with legal and ethical standards in research and experimentation.