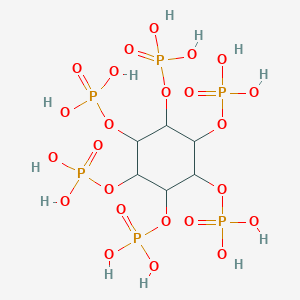
Phytic acid
Overview
Description
Phytic acid (C₆H₁₈O₂₄P₆), also known as myo-inositol hexakisphosphate, is a naturally occurring compound in plant seeds, grains, and legumes, constituting 1–5% of their dry weight . It serves as the primary storage form of phosphorus in plants, with 60–90% of total seed phosphorus bound to this compound . Structurally, it consists of a myo-inositol ring esterified with six phosphate groups (Figure 1A), enabling strong chelation of multivalent cations like Ca²⁺, Fe³⁺, and Zn²⁺ .
This compound exhibits dual roles in nutrition and industry. While it is an anti-nutrient that reduces mineral bioavailability in monogastric animals and humans , it also demonstrates antioxidant, anti-inflammatory, and antimicrobial properties . Its applications span biomedicine (e.g., bone regeneration ), food preservation , and mineral processing (e.g., selective depressant in flotation ).
Preparation Methods
Cation Exchange Resin-Based Preparation
The cation exchange method is widely employed for converting insoluble phytin (calcium-magnesium phytate) into soluble phytic acid. As detailed in US Patent 2,718,523 , the process begins by slurrying phytin in water and treating it with a hydrogen-cycle cation exchange resin. The resin displaces calcium and magnesium ions, reducing the solution’s pH to 2–3. Subsequent heating to ≥150°F (65.5°C) for ≥10 minutes precipitates residual metal phosphates, which are removed by filtration. Decolorization with activated carbon and vacuum concentration yield a syrupy this compound solution.
For large-scale production, the patent recommends passing the phytin slurry through a resin column instead of batch-wise addition. This approach improves resin utilization efficiency and produces a strongly acidic solution (pH <1.5) . Neutralization with bases like sodium hydroxide or ammonia generates soluble phytate salts. Methanol precipitation crystallizes these salts, with the final product containing ≤0.5% residual metals.
Acid Extraction and Alkaline Precipitation from Raw Materials
This compound is industrially extracted from rice bran, wheat bran, and defatted oilseed meals using dilute mineral or organic acids. US Patent 3,591,665 specifies optimal conditions:
-
Acid concentration : 0.2–2.0% (w/v) hydrochloric, sulfuric, or acetic acid
-
Solid-to-solvent ratio : 1:5–1:30 (raw material:acid solution)
-
Extraction time : 30 minutes to 5 hours at 25–55°C
After filtration, the acidic extract is neutralized to pH 9.0 with NaOH, KOH, or NH₄OH, precipitating phytin as a sodium/ammonium-calcium-magnesium complex. Washing and drying this precipitate yields phytin with 18–21% organic phosphorus content .
Table 1: Comparative Extraction Efficiency Across Acid Types
Acid Type | Concentration (M) | Extraction Yield (%) | Source |
---|---|---|---|
Hydrochloric | 1.0 | 87 | Han (1988) |
Sulfuric | 0.01 | 67 | Han (1988) |
Acetic | 1.0 | 58 | Han (1988) |
Hydrochloric | 1.0 | 94 | Fuh & Chiang (2001) |
Strong acids (HCl, H₂SO₄) outperform weak acids (acetic, formic) due to enhanced phytin solubility at low pH. However, excessive acid concentrations (>1.5 M) reduce yields by promoting protein co-precipitation .
Dual Ion Exchange Purification
Post-extraction purification involves sequential treatment with cation and anion exchange resins. In the method of US Patent 3,591,665 , the phytin dispersion is first passed through a strong cation exchanger (e.g., Amberlite IR-120) to remove Ca²⁺, Mg²⁺, and NH₄⁺ ions. The resulting this compound solution (pH 0.7–1.2) is then treated with a weak anion resin (e.g., Amberlite IR-4B) to adsorb sulfate or chloride ions from the extraction acid.
Key Purification Parameters:
-
Resin bed volume : 2–3× the phytin solution volume
-
Flow rate : 10–20 mL/min
Post-resin treatment, vacuum concentration at ≤50°C and 30 mmHg produces a 70–75% this compound syrup. Yields reach 97–99% when starting from ammonium-calcium-magnesium phytin .
Solvent-Assisted Crystallization
Methanol-induced crystallization converts purified this compound solutions into stable solid forms. The US Patent 2,718,523 protocol involves:
-
Concentrating the resin-treated solution to 46° Baumé (≈80% w/w)
-
Adding the syrup to 3× volume anhydrous methanol under agitation
-
Filtering and washing crystals with cold methanol
-
Drying at 40–50°C under reduced pressure
This method yields colorless crystals with <0.1% residual solvents. This compound remains fully soluble in methanol, while impurities like metal phytates precipitate selectively .
Optimization of Extraction and Purification
Recent studies refine traditional methods by optimizing time, temperature, and resin ratios:
Table 2: Optimized Conditions for Rice Bran Extraction
Notably, prolonged extraction (>1 hour) or elevated temperatures (>40°C) degrade this compound into lower inositol phosphates, reducing yields . Post-extraction dialysis or ultrafiltration membranes (10 kDa MWCO) further improve purity to >99% by removing polysaccharides and proteins .
Quality Control and Analytical Validation
Quantitative analysis via UV/Vis spectroscopy ensures batch consistency. The Megazyme® enzymatic method involves:
-
Releasing inorganic phosphate (Pi) from this compound using phytase and alkaline phosphatase
-
Reacting Pi with ammonium molybdate to form molybdenum blue
-
Measuring absorbance at 655 nm (ε = 4,200 L·mol⁻¹·cm⁻¹)
Calibration curves linear up to 24,000 mg/kg this compound (R² >0.999) enable precise quantification. Inter-laboratory validation shows 92–99% accuracy and 4–7% RSD precision .
Chemical Reactions Analysis
Types of Reactions: Phytate undergoes various chemical reactions, including hydrolysis, dephosphorylation, and complexation. Hydrolysis of phytate is catalyzed by the enzyme phytase, which sequentially removes phosphate groups, resulting in lower inositol phosphates and free phosphate ions .
Common Reagents and Conditions:
Hydrolysis: Catalyzed by phytase enzymes under acidic conditions (pH 2.5 to 5.5) and temperatures ranging from 37°C to 55°C.
Dephosphorylation: Achieved using strong acids or enzymatic action of phytases.
Complexation: Phytate forms complexes with metal ions such as calcium, iron, and zinc under physiological pH conditions.
Major Products Formed:
Hydrolysis: Produces lower inositol phosphates (e.g., inositol pentakisphosphate, inositol tetrakisphosphate) and free phosphate ions.
Dephosphorylation: Results in the formation of inositol and inorganic phosphate.
Scientific Research Applications
Nutritional Applications
1. Mineral Absorption Modulation
Phytic acid has been shown to influence mineral absorption negatively by forming insoluble complexes with minerals such as zinc, iron, calcium, and magnesium. However, recent studies suggest that its role may be more complex than previously thought. For instance, while high dietary phytate levels can inhibit mineral absorption, moderate consumption may not significantly affect mineral status in well-nourished individuals .
2. Antioxidant Properties
this compound exhibits potent antioxidant activity due to its ability to scavenge free radicals and chelate iron, which can catalyze oxidative reactions. This property is beneficial in preventing oxidative stress-related diseases such as cancer and cardiovascular diseases .
3. Anti-Cancer Potential
Research indicates that this compound may have anti-cancer properties by inhibiting tumor growth and inducing apoptosis in cancer cells. Clinical trials have demonstrated its efficacy in reducing serum uric acid levels and potentially lowering the risk of certain cancers .
4. Dietary Supplementation
this compound is increasingly recognized as a functional ingredient in dietary supplements aimed at enhancing health outcomes. Its incorporation into diets has been linked to improvements in metabolic markers such as blood glucose and cholesterol levels .
Medical Applications
1. Treatment of Kidney Stones
this compound has been studied for its potential role in preventing the formation of kidney stones by inhibiting calcium oxalate crystallization. Animal studies have shown promising results regarding its efficacy in reducing stone formation .
2. Neuroprotective Effects
Emerging evidence suggests that this compound may exert neuroprotective effects, potentially lowering the risk of neurodegenerative diseases through its antioxidant properties and ability to modulate metal ion homeostasis .
Industrial Applications
1. Food Preservation
Due to its GRAS (Generally Recognized As Safe) status in various regions, this compound is utilized as a natural preservative in food products. Its antioxidant properties help extend shelf life by preventing rancidity and spoilage caused by oxidative degradation .
2. Heavy Metal Remediation
this compound is employed in environmental applications for the remediation of heavy metal-contaminated waste. Its chelating ability allows it to bind heavy metals, facilitating their removal from contaminated sites .
3. Flame Retardant Properties
Recent studies have explored the use of this compound as a bio-based component in textiles to impart flame-retardant properties. This application aligns with increasing demands for sustainable materials .
Clinical Trials
- Study on Uric Acid Levels : A double-blind study involving 48 healthy volunteers demonstrated that a this compound drink significantly reduced serum uric acid levels compared to a control group .
- Impact on Cancer Cells : Research conducted on various cancer cell lines revealed that this compound inhibited cell proliferation and induced apoptosis, suggesting its potential as an adjunct therapy in cancer treatment .
Environmental Impact
Mechanism of Action
Phytate exerts its effects primarily through its strong binding affinity to metal ions. By chelating metal ions such as calcium, iron, and zinc, phytate can inhibit their absorption in the digestive system. This chelation process also contributes to its antioxidant properties by reducing iron-related free radical generation. Additionally, phytate’s ability to inhibit calcium salt crystallization helps prevent the formation of kidney stones and vascular calcifications .
Comparison with Similar Compounds
Chelating Agents: Phytic Acid vs. EDTA and Sodium Tripolyphosphate
This compound outperforms synthetic chelators like EDTA and sodium tripolyphosphate in certain applications due to its natural origin and multi-dentate binding capacity.
In water-softening experiments, this compound achieved superior Ca²⁺ removal (98%) compared to EDTA (85%) and sodium tripolyphosphate (90%) under neutral pH conditions . Its multivalent binding also enhances mechanical properties in hydrogels, outperforming monovalent ions .
Antioxidants: this compound vs. Phenolic Compounds
While this compound shows antioxidant activity, it is less effective than phenolic compounds in radical scavenging:
In dehusked rice, this compound contributed minimally to DPPH scavenging and reducing power, whereas phenolic compounds dominated antioxidant effects . However, this compound excels in inhibiting iron-catalyzed lipid oxidation, a property leveraged in food preservation .
Inositol Phosphates: this compound vs. Lower Phosphorylated Forms
This compound (IP6) is hydrolyzed by phytase into lower inositol phosphates (IP1–IP5), which differ in bioavailability and bioactivity:
Phytase from Aspergillus niger hydrolyzes this compound 50× faster than IP5 or IP4 .
Phosphate Sources: this compound vs. Inorganic Phosphates
This compound’s anti-nutritional effects contrast with inorganic phosphates (e.g., monocalcium phosphate) in animal feed:
Supplementation with microbial phytase improves this compound’s phosphorus bioavailability to 70%, matching inorganic phosphates .
Biological Activity
Phytic acid, or myo-inositol hexakisphosphate (IP6), is a naturally occurring compound found predominantly in seeds, grains, and legumes. It plays a significant role in various biological activities, including its functions as an antioxidant, its impact on mineral absorption, and its potential therapeutic applications. This article delves into the biological activities of this compound, supported by diverse research findings and case studies.
1. Antioxidant Properties
This compound exhibits notable antioxidant properties, which contribute to its health benefits. Research indicates that it can scavenge free radicals and reduce oxidative stress, potentially lowering the risk of chronic diseases such as cancer and cardiovascular diseases .
Case Study: Anticancer Potential
A study by Vucenik and Shamsuddin (2006) highlighted this compound's anticancer properties, showing that it could inhibit tumor growth in animal models. The mechanism involves the modulation of cellular signaling pathways related to apoptosis and cell cycle regulation .
2. Mineral Absorption Inhibition
Despite its health benefits, this compound is often labeled as an "anti-nutritional factor" due to its ability to chelate essential minerals such as calcium, iron, zinc, and magnesium. This chelation can significantly inhibit their absorption in the gastrointestinal tract .
Table 1: Impact of this compound on Mineral Absorption
Mineral | Effect on Absorption (%) | Reference |
---|---|---|
Calcium | Decreased by 45% | Kim et al. (2007) |
Iron | Decreased by 33% | Kim et al. (1993) |
Zinc | Decreased by 18% | Rubio et al. (1994) |
3. Health Benefits and Therapeutic Applications
Recent studies have explored the potential health benefits of this compound beyond its role as an anti-nutritional factor:
- Diabetes Management : A randomized clinical trial by Sanchis et al. (2018) demonstrated that a diet high in phytates could lower levels of advanced glycation end-products (AGEs) and glycated hemoglobin (HbA1c) in type 2 diabetes patients .
- Uric Acid Regulation : Ikenaga et al. (2020) found that consumption of a this compound drink significantly reduced serum uric acid levels in healthy volunteers, suggesting a role for this compound in managing conditions like gout .
Table 2: Clinical Trials Involving this compound
Study | Population | Intervention | Outcome |
---|---|---|---|
Proietti et al. (2017) | Breast cancer patients | 200 mg/day phytate | Improved quality of life |
Sanchis et al. (2018) | Type 2 diabetes patients | Phytate-based diet | Reduced HbA1c and AGEs |
Ikenaga et al. (2020) | Healthy volunteers | This compound drink (600 mg) | Decreased serum uric acid levels |
This compound's biological activity is largely attributed to its ability to interact with proteins and minerals:
- Protein Interaction : this compound can bind to cationic amino acids in proteins, influencing protein digestion and bioavailability . While this can be detrimental by reducing nutrient absorption, it may also protect against harmful proteins.
- Microbial Interactions : Certain gut microbiota possess phytase enzymes that can degrade this compound into bioavailable forms of phosphate and inositol, enhancing mineral absorption post-fermentation .
5. Conclusion
This compound serves as a double-edged sword in nutrition and health—while it inhibits the absorption of essential minerals, it also offers significant health benefits through its antioxidant properties and potential therapeutic effects against chronic diseases such as cancer and diabetes. Future research should continue to explore these dual roles, particularly focusing on dietary strategies that maximize the benefits of this compound while mitigating its anti-nutritional effects.
Q & A
Basic Research Questions
Q. What are the standard methodologies for quantifying phytic acid in plant-based samples, and how do they address interference from other antinutrients?
this compound quantification often employs colorimetric assays (e.g., Wade reagent) or HPLC, with modifications to mitigate interference from tannins, oxalates, or inorganic phosphates. For example, the Davies and Reid (1979) method involves acid extraction followed by spectrophotometric analysis, adjusted with chelating agents to isolate this compound . Pre-treatment steps, such as enzymatic hydrolysis of tannins, are critical to reduce cross-reactivity. Always validate methods using spike-and-recovery experiments to confirm specificity.
Q. How can researchers design experiments to assess this compound’s impact on mineral bioavailability in in vitro models?
Use simulated gastrointestinal digestion protocols (e.g., INFOGEST) with controlled pH and enzyme conditions. Measure mineral solubility (e.g., Fe, Zn) via ICP-MS before and after this compound exposure. Include controls with phytase enzyme treatment to isolate this compound’s effects. Replicates should account for matrix effects from sample types (e.g., biofortified vs. conventional crops) .
Q. What statistical approaches are recommended for analyzing variability in this compound content across plant genotypes?
Apply ANOVA to compare means across genotypes, followed by post-hoc tests (e.g., LSD) to identify significant differences. Use Pearson correlation to explore relationships between this compound and micronutrients (e.g., Fe/Zn). For large datasets, multivariate analyses (PCA) can reveal clustering patterns .
Advanced Research Questions
Q. How can experimental models resolve contradictory findings about this compound’s dual roles as an antinutrient and antioxidant?
Adopt in vivo and in vitro parallel studies:
- In vitro: Measure antioxidant capacity (e.g., DPPH assay) under varying pH and redox conditions.
- In vivo (animal models): Compare biomarkers of oxidative stress (e.g., MDA levels) and mineral status in diets with/without this compound.
Context-dependent effects often arise from dosage and interaction with dietary components (e.g., ascorbic acid), necessitating factorial experimental designs .
Q. What strategies mitigate confounding factors when studying this compound inactivation in legume processing (e.g., soaking, germination)?
- Controlled variables : Standardize temperature, duration, and seed batch.
- This compound tracking : Use time-series sampling to monitor degradation kinetics.
- Nutrient trade-offs : Simultaneously quantify phytate reduction and micronutrient losses (e.g., thiamine) to evaluate net nutritional gain .
- Replication : Include biological and technical replicates to account for natural variability in seed this compound content .
Q. How can researchers address the lack of consensus on phosphorus reallocation mechanisms in low-phytate plant mutants?
Combine isotopic labeling (e.g., ³²P) with metabolomic profiling to trace phosphorus flux in mutants. Compare phosphorus partitioning in roots, shoots, and grains. Use RNA-seq to identify differentially expressed genes in phosphorus transport pathways. Validate findings via CRISPR knockouts in model plants (e.g., Arabidopsis) .
Q. What experimental frameworks are optimal for evaluating this compound’s role in plant stress resistance?
- Abiotic stress : Expose plants to drought or salinity, measuring this compound accumulation and stress markers (e.g., proline).
- Biotic stress : Infect plants with pathogens and quantify this compound in infected vs. healthy tissues.
- Mechanistic studies : Use mutants with altered this compound biosynthesis to isolate its contribution to stress adaptation .
Q. Methodological Best Practices
- Reproducibility : Document extraction protocols, instrument parameters (e.g., HPLC column type), and calibration curves in supplementary materials .
- Data contradictions : Perform sensitivity analyses to identify outlier-prone steps (e.g., extraction pH variability) .
- Ethical considerations : For animal studies, justify this compound dosages based on FAO/WHO guidelines to avoid non-physiological exposures .
Properties
IUPAC Name |
(2,3,4,5,6-pentaphosphonooxycyclohexyl) dihydrogen phosphate | |
---|---|---|
Source | PubChem | |
URL | https://pubchem.ncbi.nlm.nih.gov | |
Description | Data deposited in or computed by PubChem | |
InChI |
InChI=1S/C6H18O24P6/c7-31(8,9)25-1-2(26-32(10,11)12)4(28-34(16,17)18)6(30-36(22,23)24)5(29-35(19,20)21)3(1)27-33(13,14)15/h1-6H,(H2,7,8,9)(H2,10,11,12)(H2,13,14,15)(H2,16,17,18)(H2,19,20,21)(H2,22,23,24) | |
Source | PubChem | |
URL | https://pubchem.ncbi.nlm.nih.gov | |
Description | Data deposited in or computed by PubChem | |
InChI Key |
IMQLKJBTEOYOSI-UHFFFAOYSA-N | |
Source | PubChem | |
URL | https://pubchem.ncbi.nlm.nih.gov | |
Description | Data deposited in or computed by PubChem | |
Canonical SMILES |
C1(C(C(C(C(C1OP(=O)(O)O)OP(=O)(O)O)OP(=O)(O)O)OP(=O)(O)O)OP(=O)(O)O)OP(=O)(O)O | |
Source | PubChem | |
URL | https://pubchem.ncbi.nlm.nih.gov | |
Description | Data deposited in or computed by PubChem | |
Molecular Formula |
C6H18O24P6 | |
Source | PubChem | |
URL | https://pubchem.ncbi.nlm.nih.gov | |
Description | Data deposited in or computed by PubChem | |
DSSTOX Substance ID |
DTXSID40889331, DTXSID00861653 | |
Record name | myo-Inositol hexakisphosphate | |
Source | EPA DSSTox | |
URL | https://comptox.epa.gov/dashboard/DTXSID40889331 | |
Description | DSSTox provides a high quality public chemistry resource for supporting improved predictive toxicology. | |
Record name | Cyclohexane-1,2,3,4,5,6-hexayl hexakis[dihydrogen (phosphate)] | |
Source | EPA DSSTox | |
URL | https://comptox.epa.gov/dashboard/DTXSID00861653 | |
Description | DSSTox provides a high quality public chemistry resource for supporting improved predictive toxicology. | |
Molecular Weight |
660.04 g/mol | |
Source | PubChem | |
URL | https://pubchem.ncbi.nlm.nih.gov | |
Description | Data deposited in or computed by PubChem | |
Physical Description |
Straw-colored syrupy liquid; [Merck Index] | |
Record name | Phytic acid | |
Source | Haz-Map, Information on Hazardous Chemicals and Occupational Diseases | |
URL | https://haz-map.com/Agents/21491 | |
Description | Haz-Map® is an occupational health database designed for health and safety professionals and for consumers seeking information about the adverse effects of workplace exposures to chemical and biological agents. | |
Explanation | Copyright (c) 2022 Haz-Map(R). All rights reserved. Unless otherwise indicated, all materials from Haz-Map are copyrighted by Haz-Map(R). No part of these materials, either text or image may be used for any purpose other than for personal use. Therefore, reproduction, modification, storage in a retrieval system or retransmission, in any form or by any means, electronic, mechanical or otherwise, for reasons other than personal use, is strictly prohibited without prior written permission. | |
CAS No. |
83-86-3 | |
Record name | Phytic acid | |
Source | ChemIDplus | |
URL | https://pubchem.ncbi.nlm.nih.gov/substance/?source=chemidplus&sourceid=0000083863 | |
Description | ChemIDplus is a free, web search system that provides access to the structure and nomenclature authority files used for the identification of chemical substances cited in National Library of Medicine (NLM) databases, including the TOXNET system. | |
Record name | Phytic acid | |
Source | DrugBank | |
URL | https://www.drugbank.ca/drugs/DB14981 | |
Description | The DrugBank database is a unique bioinformatics and cheminformatics resource that combines detailed drug (i.e. chemical, pharmacological and pharmaceutical) data with comprehensive drug target (i.e. sequence, structure, and pathway) information. | |
Explanation | Creative Common's Attribution-NonCommercial 4.0 International License (http://creativecommons.org/licenses/by-nc/4.0/legalcode) | |
Record name | Phytic acid | |
Source | DTP/NCI | |
URL | https://dtp.cancer.gov/dtpstandard/servlet/dwindex?searchtype=NSC&outputformat=html&searchlist=269896 | |
Description | The NCI Development Therapeutics Program (DTP) provides services and resources to the academic and private-sector research communities worldwide to facilitate the discovery and development of new cancer therapeutic agents. | |
Explanation | Unless otherwise indicated, all text within NCI products is free of copyright and may be reused without our permission. Credit the National Cancer Institute as the source. | |
Record name | myo-Inositol, 1,2,3,4,5,6-hexakis(dihydrogen phosphate) | |
Source | EPA Chemicals under the TSCA | |
URL | https://www.epa.gov/chemicals-under-tsca | |
Description | EPA Chemicals under the Toxic Substances Control Act (TSCA) collection contains information on chemicals and their regulations under TSCA, including non-confidential content from the TSCA Chemical Substance Inventory and Chemical Data Reporting. | |
Record name | myo-Inositol hexakisphosphate | |
Source | EPA DSSTox | |
URL | https://comptox.epa.gov/dashboard/DTXSID40889331 | |
Description | DSSTox provides a high quality public chemistry resource for supporting improved predictive toxicology. | |
Record name | Fytic acid | |
Source | European Chemicals Agency (ECHA) | |
URL | https://echa.europa.eu/substance-information/-/substanceinfo/100.001.369 | |
Description | The European Chemicals Agency (ECHA) is an agency of the European Union which is the driving force among regulatory authorities in implementing the EU's groundbreaking chemicals legislation for the benefit of human health and the environment as well as for innovation and competitiveness. | |
Explanation | Use of the information, documents and data from the ECHA website is subject to the terms and conditions of this Legal Notice, and subject to other binding limitations provided for under applicable law, the information, documents and data made available on the ECHA website may be reproduced, distributed and/or used, totally or in part, for non-commercial purposes provided that ECHA is acknowledged as the source: "Source: European Chemicals Agency, http://echa.europa.eu/". Such acknowledgement must be included in each copy of the material. ECHA permits and encourages organisations and individuals to create links to the ECHA website under the following cumulative conditions: Links can only be made to webpages that provide a link to the Legal Notice page. | |
Record name | FYTIC ACID | |
Source | FDA Global Substance Registration System (GSRS) | |
URL | https://gsrs.ncats.nih.gov/ginas/app/beta/substances/7IGF0S7R8I | |
Description | The FDA Global Substance Registration System (GSRS) enables the efficient and accurate exchange of information on what substances are in regulated products. Instead of relying on names, which vary across regulatory domains, countries, and regions, the GSRS knowledge base makes it possible for substances to be defined by standardized, scientific descriptions. | |
Explanation | Unless otherwise noted, the contents of the FDA website (www.fda.gov), both text and graphics, are not copyrighted. They are in the public domain and may be republished, reprinted and otherwise used freely by anyone without the need to obtain permission from FDA. Credit to the U.S. Food and Drug Administration as the source is appreciated but not required. | |
Synthesis routes and methods I
Procedure details
Synthesis routes and methods II
Procedure details
Synthesis routes and methods III
Procedure details
Synthesis routes and methods IV
Procedure details
Retrosynthesis Analysis
AI-Powered Synthesis Planning: Our tool employs the Template_relevance Pistachio, Template_relevance Bkms_metabolic, Template_relevance Pistachio_ringbreaker, Template_relevance Reaxys, Template_relevance Reaxys_biocatalysis model, leveraging a vast database of chemical reactions to predict feasible synthetic routes.
One-Step Synthesis Focus: Specifically designed for one-step synthesis, it provides concise and direct routes for your target compounds, streamlining the synthesis process.
Accurate Predictions: Utilizing the extensive PISTACHIO, BKMS_METABOLIC, PISTACHIO_RINGBREAKER, REAXYS, REAXYS_BIOCATALYSIS database, our tool offers high-accuracy predictions, reflecting the latest in chemical research and data.
Strategy Settings
Precursor scoring | Relevance Heuristic |
---|---|
Min. plausibility | 0.01 |
Model | Template_relevance |
Template Set | Pistachio/Bkms_metabolic/Pistachio_ringbreaker/Reaxys/Reaxys_biocatalysis |
Top-N result to add to graph | 6 |
Feasible Synthetic Routes
Disclaimer and Information on In-Vitro Research Products
Please be aware that all articles and product information presented on BenchChem are intended solely for informational purposes. The products available for purchase on BenchChem are specifically designed for in-vitro studies, which are conducted outside of living organisms. In-vitro studies, derived from the Latin term "in glass," involve experiments performed in controlled laboratory settings using cells or tissues. It is important to note that these products are not categorized as medicines or drugs, and they have not received approval from the FDA for the prevention, treatment, or cure of any medical condition, ailment, or disease. We must emphasize that any form of bodily introduction of these products into humans or animals is strictly prohibited by law. It is essential to adhere to these guidelines to ensure compliance with legal and ethical standards in research and experimentation.