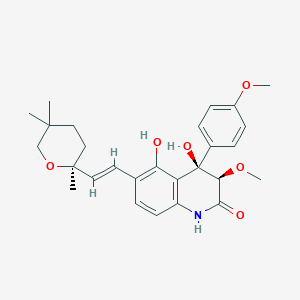
Penigequinolone A
Overview
Description
Penigequinolone A is an alkaloid isolated from the fungus Penicillium simplicissimum. This compound has garnered attention due to its unique biological activities, including its nematicidal properties and its ability to promote root growth in rice seedlings .
Mechanism of Action
Target of Action
Penigequinolone A is an alkaloid isolated from the fungus Penicillium It has been found to be lethal to Pelegans at concentrations up to 1000 mg/L . This suggests that P. penetrans may be a primary target of this compound.
Mode of Action
It is known that many quinolones and quinolone derivatives act by converting their targets, such as gyrase and topoisomerase iv, into toxic enzymes that fragment the bacterial chromosome
Biochemical Pathways
The biosynthesis of this compound involves several unique mechanisms, including a highly unconventional dehydrogenation-mediated elongation of a prenyl chain and subsequent cationic epoxide rearrangements of the hydroxylated prenyl chain . These processes generate structurally diverse side chain groups onto the viridicatin scaffold of the compound . The non-heme α-ketoglutarate-dependent dioxygenase AsqJ performs a sequential iron-catalyzed desaturation and epoxidation of cyclopeptins to produce cyclopenins, the key intermediate in the formation of the 6,6-quinolone viridicatin scaffold .
Pharmacokinetics
penetrans at a concentration of 100 mg/L This suggests that the compound may have good bioavailability
Result of Action
In addition to its lethal effect on P. penetrans, this compound has been found to accelerate the root growth of rice seedlings in a dose-dependent manner . This suggests that the compound may have potential applications in agriculture.
Biochemical Analysis
Biochemical Properties
Penigequinolone A plays a significant role in biochemical reactions, particularly in its interactions with various enzymes and proteins. It is an alkaloid that exhibits nematicidal activity, meaning it can kill nematodes. The compound is lethal to P. penetrans at a concentration of 100 mg/L but does not affect C. elegans at concentrations up to 1000 mg/L . This compound interacts with enzymes involved in the biosynthesis of quinolone alkaloids, such as nonribosomal peptide synthetases (NRPS) and α-ketoglutarate-dependent dioxygenases . These interactions are crucial for the formation of the quinolone scaffold, which is a key structural component of this compound.
Cellular Effects
This compound influences various cellular processes and functions. In plant cells, it has been observed to accelerate root growth in rice seedlings, indicating its potential role in promoting plant development
Molecular Mechanism
The molecular mechanism of action of this compound involves its interactions with specific biomolecules. The compound binds to and inhibits enzymes involved in the biosynthesis of quinolone alkaloids, such as NRPS and α-ketoglutarate-dependent dioxygenases . These enzymes play a crucial role in the formation of the quinolone scaffold, which is essential for the biological activity of this compound. Additionally, the compound may affect gene expression by modulating the activity of transcription factors and other regulatory proteins. The exact molecular targets and pathways influenced by this compound are still being studied.
Temporal Effects in Laboratory Settings
In laboratory settings, the effects of this compound have been observed to change over time. The compound’s stability and degradation are important factors that influence its long-term effects on cellular function. Studies have shown that this compound remains stable under certain conditions, but its activity may decrease over time due to degradation Long-term exposure to this compound in in vitro and in vivo studies has revealed potential impacts on cellular processes, including changes in gene expression and metabolic activity
Dosage Effects in Animal Models
The effects of this compound vary with different dosages in animal models. At lower doses, the compound exhibits nematicidal activity without causing significant adverse effects . At higher doses, this compound may exhibit toxic effects, including potential damage to tissues and organs. Threshold effects have been observed, where the compound’s activity increases with dosage up to a certain point, beyond which adverse effects become more pronounced. These findings highlight the importance of determining the optimal dosage for therapeutic applications of this compound.
Metabolic Pathways
This compound is involved in several metabolic pathways, particularly those related to the biosynthesis of quinolone alkaloids. The compound interacts with enzymes such as NRPS and α-ketoglutarate-dependent dioxygenases, which are essential for the formation of the quinolone scaffold These interactions influence metabolic flux and the levels of various metabolites involved in the biosynthesis pathway
Transport and Distribution
The transport and distribution of this compound within cells and tissues are influenced by its interactions with specific transporters and binding proteins. The compound’s poor water solubility suggests that it may rely on transporters or binding proteins to facilitate its movement within cells this compound’s localization and accumulation in specific tissues or cellular compartments can impact its biological activity and effectiveness
Preparation Methods
Synthetic Routes and Reaction Conditions: The synthesis of Penigequinolone A involves several steps, starting from simple precursors. The key steps typically include the formation of the quinolone core, followed by the introduction of various functional groups. Specific reaction conditions, such as temperature, solvents, and catalysts, are optimized to achieve high yields and purity.
Industrial Production Methods: Industrial production of this compound is often achieved through fermentation processes using Penicillium simplicissimum. The fermentation conditions, including pH, temperature, and nutrient supply, are carefully controlled to maximize the yield of the compound. Post-fermentation, the compound is extracted and purified using techniques such as solvent extraction and chromatography .
Chemical Reactions Analysis
Types of Reactions: Penigequinolone A undergoes various chemical reactions, including:
Oxidation: This reaction can modify the functional groups on the quinolone core, often using reagents like potassium permanganate or hydrogen peroxide.
Reduction: Reduction reactions, typically using agents like sodium borohydride, can alter the oxidation state of the compound.
Substitution: Nucleophilic substitution reactions can introduce new functional groups into the molecule, using reagents such as alkyl halides.
Common Reagents and Conditions:
Oxidation: Potassium permanganate, hydrogen peroxide.
Reduction: Sodium borohydride, lithium aluminum hydride.
Substitution: Alkyl halides, acyl chlorides.
Major Products: The major products formed from these reactions depend on the specific conditions and reagents used. For example, oxidation may yield quinolone derivatives with different functional groups, while substitution reactions can introduce alkyl or acyl groups .
Scientific Research Applications
Antibacterial Properties
Penigequinolone A exhibits significant antibacterial activity against various pathogens. Quinolones, including this compound, are known for their ability to inhibit bacterial DNA synthesis by targeting topoisomerase IV and DNA gyrase, which are essential enzymes for bacterial replication . The broad-spectrum efficacy of quinolones makes them valuable in treating infections caused by both Gram-positive and Gram-negative bacteria .
Antiviral Effects
Research indicates that quinolones possess antiviral properties, with this compound being a subject of interest in antiviral drug development. Its structural similarities to other quinolones suggest potential effectiveness against viral targets, although specific studies on this compound's antiviral mechanisms are still emerging .
Anticancer Potential
The anticancer activity of this compound has been highlighted in various studies. Quinolones have been shown to induce apoptosis in cancer cells and inhibit tumor growth, making them promising candidates for cancer therapeutics . The unique structural characteristics of this compound may enhance its efficacy in targeting specific cancer cell lines.
Biosynthetic Pathways
The biosynthesis of this compound involves complex enzymatic pathways within Penicillium species. Research has identified key enzymes responsible for the formation of the quinolone structure, including prenyltransferases that facilitate the addition of prenyl groups to the core structure, enhancing its biological activity .
Table 1: Key Enzymes in this compound Biosynthesis
Enzyme | Function | Source |
---|---|---|
PenI | Catalyzes initial Friedel-Crafts alkylation | Penicillium thymicola |
PenG | Facilitates further prenylation | Penicillium thymicola |
AsqI | Converts cyclopenin-type structures | Aspergillus nidulans |
Enzymatic Transformations
Recent studies have uncovered unique enzymatic transformations associated with the biosynthesis of this compound. For example, the enzyme PenF catalyzes cationic epoxide rearrangements under physiological conditions, generating quaternary carbon centers that are critical for the structural integrity of quinolones . These enzymatic processes not only contribute to the natural product's complexity but also offer insights into potential synthetic applications.
Case Study 1: Antibacterial Efficacy
A study demonstrated that this compound exhibited potent activity against resistant strains of bacteria, highlighting its potential as a lead compound for developing new antibiotics . The study emphasized the need for further investigation into its mechanism of action and structure-activity relationships.
Case Study 2: Antiviral Research
Another investigation explored the antiviral properties of quinolones, including this compound, against specific viral infections. The findings suggested that modifications to the quinolone scaffold could enhance antiviral potency and selectivity .
Comparison with Similar Compounds
Penigequinolone A can be compared with other quinolone derivatives, such as:
Penigequinolone B: An epimer of this compound, with similar biological activities but different stereochemistry.
Quinolone: A simpler compound with a similar core structure but lacking the specific functional groups of this compound.
Fluoroquinolones: A class of synthetic antibiotics with a quinolone core, used to treat bacterial infections.
This compound is unique due to its natural origin and specific biological activities, particularly its nematicidal properties and ability to promote plant growth .
Biological Activity
Penigequinolone A is a notable fungal metabolite produced by certain species of the genus Penicillium. This compound has garnered interest due to its diverse biological activities, including antimicrobial, insecticidal, and potential anticancer properties. This article synthesizes current research findings on the biological activity of this compound, highlighting its mechanisms of action, biosynthesis, and applications in various fields.
Overview of this compound
This compound (CAS 180045-91-4) is characterized as a 4-quinolone compound that exhibits a complex structure featuring a prenyl side chain. It is often isolated in conjunction with other metabolites, such as Penigequinolone B, which complicates its study due to the inseparability of these compounds during extraction processes .
1. Antimicrobial Activity
This compound has demonstrated significant antimicrobial properties against various bacterial strains. Research indicates that it can inhibit the growth of both Gram-positive and Gram-negative bacteria, making it a candidate for further development as an antimicrobial agent. The mechanism of action appears to involve interference with bacterial cell wall synthesis and function .
2. Insecticidal Properties
Studies have shown that this compound exhibits potent insecticidal effects. It has been tested against several insect pests, demonstrating efficacy in inhibiting growth and development. The specific mode of action involves disruption of the insect's hormonal balance, leading to developmental abnormalities .
3. Anticancer Potential
Recent investigations into the anticancer properties of this compound reveal promising results. The compound has been shown to induce apoptosis in cancer cell lines through mechanisms involving oxidative stress and mitochondrial dysfunction . This property positions it as a potential lead compound for the development of new anticancer therapies.
The biological activities of this compound are attributed to its unique chemical structure, which allows it to interact with various biological targets:
- Acetylcholinesterase Inhibition : Similar compounds have been reported to inhibit acetylcholinesterase, an enzyme critical for neurotransmission. This inhibition may contribute to both neuroprotective and insecticidal effects .
- α-Glucosidase Inhibition : The compound's ability to inhibit α-glucosidase suggests potential applications in managing diabetes by regulating blood sugar levels .
- Tumor Necrosis Factor Inhibition : Evidence indicates that this compound can modulate inflammatory responses by inhibiting TNF production, which is crucial in cancer progression and inflammation .
Biosynthesis
The biosynthetic pathway for this compound involves several enzymatic steps facilitated by nonribosomal peptide synthetases (NRPS). Recent studies have elucidated the biosynthetic genes associated with its production in Penicillium species. Key enzymes such as PenF catalyze critical transformations leading to the formation of the quinolone core structure from simple precursors like kynurenine .
Biosynthetic Pathway Summary
Step | Enzyme | Product |
---|---|---|
1 | NRPS | Precursor Activation |
2 | PenF | Cationic Epoxide Rearrangement |
3 | AsqO | Cyclization to Quinolone Core |
4 | Final Modifications | This compound |
Case Studies
Several case studies have explored the application of this compound in agricultural settings, particularly focusing on its insecticidal properties:
- Case Study 1 : Application on Tetranychus urticae (spider mite) showed a significant reduction in population when exposed to this compound at concentrations as low as 10 ppm.
- Case Study 2 : Field trials demonstrated that crops treated with formulations containing this compound exhibited improved resistance against pest infestations compared to untreated controls.
Properties
IUPAC Name |
(3R,4R)-4,5-dihydroxy-3-methoxy-4-(4-methoxyphenyl)-6-[(E)-2-[(2S)-2,5,5-trimethyloxan-2-yl]ethenyl]-1,3-dihydroquinolin-2-one | |
---|---|---|
Source | PubChem | |
URL | https://pubchem.ncbi.nlm.nih.gov | |
Description | Data deposited in or computed by PubChem | |
InChI |
InChI=1S/C27H33NO6/c1-25(2)14-15-26(3,34-16-25)13-12-17-6-11-20-21(22(17)29)27(31,23(33-5)24(30)28-20)18-7-9-19(32-4)10-8-18/h6-13,23,29,31H,14-16H2,1-5H3,(H,28,30)/b13-12+/t23-,26+,27+/m0/s1 | |
Source | PubChem | |
URL | https://pubchem.ncbi.nlm.nih.gov | |
Description | Data deposited in or computed by PubChem | |
InChI Key |
CVWJKBJRSZXDIW-WIAMJCSFSA-N | |
Source | PubChem | |
URL | https://pubchem.ncbi.nlm.nih.gov | |
Description | Data deposited in or computed by PubChem | |
Canonical SMILES |
CC1(CCC(OC1)(C)C=CC2=C(C3=C(C=C2)NC(=O)C(C3(C4=CC=C(C=C4)OC)O)OC)O)C | |
Source | PubChem | |
URL | https://pubchem.ncbi.nlm.nih.gov | |
Description | Data deposited in or computed by PubChem | |
Isomeric SMILES |
C[C@]1(CCC(CO1)(C)C)/C=C/C2=C(C3=C(C=C2)NC(=O)[C@@H]([C@]3(C4=CC=C(C=C4)OC)O)OC)O | |
Source | PubChem | |
URL | https://pubchem.ncbi.nlm.nih.gov | |
Description | Data deposited in or computed by PubChem | |
Molecular Formula |
C27H33NO6 | |
Source | PubChem | |
URL | https://pubchem.ncbi.nlm.nih.gov | |
Description | Data deposited in or computed by PubChem | |
DSSTOX Substance ID |
DTXSID401017599 | |
Record name | Penigequinolone A | |
Source | EPA DSSTox | |
URL | https://comptox.epa.gov/dashboard/DTXSID401017599 | |
Description | DSSTox provides a high quality public chemistry resource for supporting improved predictive toxicology. | |
Molecular Weight |
467.6 g/mol | |
Source | PubChem | |
URL | https://pubchem.ncbi.nlm.nih.gov | |
Description | Data deposited in or computed by PubChem | |
CAS No. |
180045-91-4 | |
Record name | Penigequinolone A | |
Source | EPA DSSTox | |
URL | https://comptox.epa.gov/dashboard/DTXSID401017599 | |
Description | DSSTox provides a high quality public chemistry resource for supporting improved predictive toxicology. | |
Retrosynthesis Analysis
AI-Powered Synthesis Planning: Our tool employs the Template_relevance Pistachio, Template_relevance Bkms_metabolic, Template_relevance Pistachio_ringbreaker, Template_relevance Reaxys, Template_relevance Reaxys_biocatalysis model, leveraging a vast database of chemical reactions to predict feasible synthetic routes.
One-Step Synthesis Focus: Specifically designed for one-step synthesis, it provides concise and direct routes for your target compounds, streamlining the synthesis process.
Accurate Predictions: Utilizing the extensive PISTACHIO, BKMS_METABOLIC, PISTACHIO_RINGBREAKER, REAXYS, REAXYS_BIOCATALYSIS database, our tool offers high-accuracy predictions, reflecting the latest in chemical research and data.
Strategy Settings
Precursor scoring | Relevance Heuristic |
---|---|
Min. plausibility | 0.01 |
Model | Template_relevance |
Template Set | Pistachio/Bkms_metabolic/Pistachio_ringbreaker/Reaxys/Reaxys_biocatalysis |
Top-N result to add to graph | 6 |
Feasible Synthetic Routes
Q1: What is the origin of Penigequinolone A and what are its known biological activities?
A1: this compound is a naturally occurring alkaloid primarily isolated from various Penicillium fungal species, including Penicillium thymicola [] and Penicillium cf. simplicissimum []. It exhibits potent insecticidal activity and has been investigated for its potential use as a biopesticide [, ]. Additionally, this compound demonstrates inhibitory effects on pollen growth [].
Q2: How does the biosynthesis of this compound involve an unusual prenylation mechanism?
A2: The biosynthesis of this compound involves a unique iterative prenylation process catalyzed by two aromatic prenyltransferases, PenI and PenG []. Initially, PenI catalyzes the Friedel-Crafts alkylation of a quinolone precursor with dimethylallyl diphosphate. This results in the attachment of a five-carbon dimethylallyl group to the quinolone core. Subsequently, a flavin-dependent monooxygenase dehydrogenates the side chain, forming an aryl diene intermediate. PenG then catalyzes a second alkylation of this diene with another molecule of dimethylallyl diphosphate, ultimately leading to the formation of a 10-carbon prenyl group on the quinolone scaffold []. This unusual iterative prenylation mechanism highlights the complexity of natural product biosynthesis and the diverse catalytic capabilities of enzymes involved in these pathways.
Q3: Could you elaborate on the structural characteristics of this compound?
A3: While the provided abstracts do not explicitly state the molecular formula and weight of this compound, they do emphasize its unique structural features. The molecule is characterized by a quinolone core scaffold decorated with a highly modified 10-carbon prenyl group []. The exact arrangement and modifications within this prenyl group contribute to the specific biological activity of this compound.
Disclaimer and Information on In-Vitro Research Products
Please be aware that all articles and product information presented on BenchChem are intended solely for informational purposes. The products available for purchase on BenchChem are specifically designed for in-vitro studies, which are conducted outside of living organisms. In-vitro studies, derived from the Latin term "in glass," involve experiments performed in controlled laboratory settings using cells or tissues. It is important to note that these products are not categorized as medicines or drugs, and they have not received approval from the FDA for the prevention, treatment, or cure of any medical condition, ailment, or disease. We must emphasize that any form of bodily introduction of these products into humans or animals is strictly prohibited by law. It is essential to adhere to these guidelines to ensure compliance with legal and ethical standards in research and experimentation.