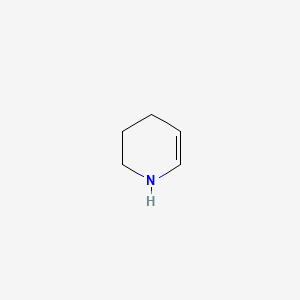
Tetrahydropyridine
Overview
Description
Tetrahydropyridine is a heterocyclic compound with the chemical formula C5H9N. It exists in three isomeric forms, which differ by the location of the double bond within the ring structure. These isomers are 1,2,3,4-tetrahydropyridine, 1,2,3,6-tetrahydropyridine, and 2,3,4,5-tetrahydropyridine . This compound is primarily of theoretical interest, as the parent compounds are not widely found in nature. many substituted derivatives of this compound are known and have significant applications in various fields .
Synthetic Routes and Reaction Conditions:
Partial Reduction of Pyridinium Salts: One common method involves the partial reduction of pyridinium salts to yield N-alkyltetrahydropyridines.
Ireland-Claisen Rearrangement: This method involves a modified Ireland-Claisen rearrangement, leading to tetrahydropyridines via a silyl ketene acetal intermediate.
Ring-Closing Olefin Metathesis: This technique has also been employed to establish the this compound ring system.
Industrial Production Methods:
Catalytic Hydrogenation: Industrially, this compound derivatives can be synthesized using catalytic hydrogenation of pyridine derivatives under specific conditions.
Multicomponent Reactions: One-pot multicomponent reactions involving various catalysts such as p-toluene sulfonic acid monohydrate, ruthenium chloride, and others have been used to synthesize this compound derivatives.
Types of Reactions:
Oxidation: this compound can undergo oxidation reactions, often leading to the formation of pyridine derivatives.
Substitution: Nucleophilic substitution reactions can be performed on this compound derivatives to introduce different functional groups.
Common Reagents and Conditions:
Oxidizing Agents: Common oxidizing agents include potassium permanganate and hydrogen peroxide.
Reducing Agents: Sodium borohydride and lithium aluminum hydride are frequently used reducing agents.
Major Products:
Pyridine Derivatives: Oxidation of this compound typically yields pyridine derivatives.
Substituted Tetrahydropyridines: Substitution reactions result in various substituted this compound compounds.
Scientific Research Applications
Tetrahydropyridine and its derivatives have a wide range of applications in scientific research:
Mechanism of Action
The mechanism of action of tetrahydropyridine derivatives varies depending on their specific structure and target:
Comparison with Similar Compounds
Tetrahydropyridine is similar to other nitrogen-containing heterocycles such as pyridine, dihydropyridine, and piperidine:
Pyridine: Pyridine is a six-membered aromatic ring with one nitrogen atom.
Dihydropyridine: Dihydropyridine is a partially saturated derivative of pyridine with two hydrogen atoms added.
Piperidine: Piperidine is a fully saturated six-membered ring with one nitrogen atom.
Uniqueness of this compound:
Isomerism: this compound exists in three isomeric forms, which is unique compared to the other similar compounds.
Biological Activity: The specific biological activities of this compound derivatives, such as their neurotoxic and enzyme inhibitory properties, distinguish them from other nitrogen-containing heterocycles.
Biological Activity
Tetrahydropyridine (THP) is a bicyclic organic compound that has garnered significant interest in pharmacology due to its diverse biological activities. This article explores the biological activity of THP, focusing on its derivatives, mechanisms of action, and implications for therapeutic applications.
Overview of this compound
This compound is characterized by a saturated six-membered ring containing five carbon atoms and one nitrogen atom. Its derivatives have been synthesized and studied extensively for their potential therapeutic effects, including anti-inflammatory, anticancer, and neuroprotective properties.
1. Anti-inflammatory Properties
THP derivatives have shown promising anti-inflammatory effects. For instance, certain N- and alpha-position substituted this compound derivatives demonstrated significant inhibition of tumor necrosis factor-alpha (TNF-α) production in vitro and in vivo. Notably, compound 3o exhibited an IC50 value of 0.44 µM against lipopolysaccharide (LPS)-induced TNF-α production in human whole blood, while compound 3i showed an ID50 of 1.42 mg/kg in mice .
Table 1: Anti-inflammatory Activity of THP Derivatives
Compound | IC50 (µM) | ID50 (mg/kg) | Activity |
---|---|---|---|
3o | 0.44 | - | In vitro inhibition of TNF-α |
3i | - | 1.42 | In vivo inhibition of TNF-α |
2. Neuroprotective Effects
The neurotoxicity associated with 1-methyl-4-phenyl-1,2,3,6-tetrahydropyridine (MPTP) is well-documented as it induces parkinsonism in humans and animal models. MPTP exposure leads to selective degeneration of dopaminergic neurons in the substantia nigra, mimicking Parkinson's disease symptoms . Research indicates that THP derivatives may counteract these neurotoxic effects through various mechanisms:
- Cox-2 Modulation : Studies have shown that MPTP treatment increases cyclooxygenase-2 (Cox-2) expression in surviving dopaminergic neurons, suggesting a role for inflammatory pathways in neurodegeneration .
- Neuroprotective Agents : Some THP derivatives have been identified as potential neuroprotective agents by inhibiting oxidative stress and inflammatory responses in neuronal cells .
3. Anticancer Activity
Recent studies have highlighted the anticancer potential of THP derivatives. For example, compounds synthesized through multicomponent reactions exhibited significant cytotoxicity against various cancer cell lines:
- Table 2: Anticancer Activity of THP Derivatives
Compound | Cell Line | IC50 (µM) |
---|---|---|
49 | SK-N-SH | 11 ± 1.3 |
50 | SK-N-SH | 22 ± 1.3 |
51 | A549 | 58 ± 4.1 |
These compounds demonstrated selective cytotoxicity, indicating their potential for further development as cancer therapeutics .
The biological activity of THP derivatives can be attributed to several mechanisms:
- Receptor Agonism : Some THP compounds act as agonists at serotonin receptors (e.g., 5-HT2C), which may contribute to their therapeutic effects in conditions like obesity and depression .
- Enzyme Inhibition : Certain derivatives have been found to inhibit acetylcholinesterase (AChE), which is crucial for managing Alzheimer's disease symptoms .
Case Studies
A notable case involved a patient with MPTP-induced parkinsonism who underwent deep brain stimulation (DBS). This case illustrated the translational potential of using MPTP as a model for studying Parkinson's disease therapies and highlighted the importance of THP-related compounds in developing neuroprotective strategies .
Properties
IUPAC Name |
1,2,3,4-tetrahydropyridine | |
---|---|---|
Source | PubChem | |
URL | https://pubchem.ncbi.nlm.nih.gov | |
Description | Data deposited in or computed by PubChem | |
InChI |
InChI=1S/C5H9N/c1-2-4-6-5-3-1/h2,4,6H,1,3,5H2 | |
Source | PubChem | |
URL | https://pubchem.ncbi.nlm.nih.gov | |
Description | Data deposited in or computed by PubChem | |
InChI Key |
VSWICNJIUPRZIK-UHFFFAOYSA-N | |
Source | PubChem | |
URL | https://pubchem.ncbi.nlm.nih.gov | |
Description | Data deposited in or computed by PubChem | |
Canonical SMILES |
C1CC=CNC1 | |
Source | PubChem | |
URL | https://pubchem.ncbi.nlm.nih.gov | |
Description | Data deposited in or computed by PubChem | |
Molecular Formula |
C5H9N | |
Source | PubChem | |
URL | https://pubchem.ncbi.nlm.nih.gov | |
Description | Data deposited in or computed by PubChem | |
DSSTOX Substance ID |
DTXSID40436364 | |
Record name | Tetrahydropyridine | |
Source | EPA DSSTox | |
URL | https://comptox.epa.gov/dashboard/DTXSID40436364 | |
Description | DSSTox provides a high quality public chemistry resource for supporting improved predictive toxicology. | |
Molecular Weight |
83.13 g/mol | |
Source | PubChem | |
URL | https://pubchem.ncbi.nlm.nih.gov | |
Description | Data deposited in or computed by PubChem | |
CAS No. |
37497-65-7 | |
Record name | 1,2,3,4-Tetrahydropyridine | |
Source | CAS Common Chemistry | |
URL | https://commonchemistry.cas.org/detail?cas_rn=37497-65-7 | |
Description | CAS Common Chemistry is an open community resource for accessing chemical information. Nearly 500,000 chemical substances from CAS REGISTRY cover areas of community interest, including common and frequently regulated chemicals, and those relevant to high school and undergraduate chemistry classes. This chemical information, curated by our expert scientists, is provided in alignment with our mission as a division of the American Chemical Society. | |
Explanation | The data from CAS Common Chemistry is provided under a CC-BY-NC 4.0 license, unless otherwise stated. | |
Record name | Tetrahydropyridine | |
Source | EPA DSSTox | |
URL | https://comptox.epa.gov/dashboard/DTXSID40436364 | |
Description | DSSTox provides a high quality public chemistry resource for supporting improved predictive toxicology. | |
Retrosynthesis Analysis
AI-Powered Synthesis Planning: Our tool employs the Template_relevance Pistachio, Template_relevance Bkms_metabolic, Template_relevance Pistachio_ringbreaker, Template_relevance Reaxys, Template_relevance Reaxys_biocatalysis model, leveraging a vast database of chemical reactions to predict feasible synthetic routes.
One-Step Synthesis Focus: Specifically designed for one-step synthesis, it provides concise and direct routes for your target compounds, streamlining the synthesis process.
Accurate Predictions: Utilizing the extensive PISTACHIO, BKMS_METABOLIC, PISTACHIO_RINGBREAKER, REAXYS, REAXYS_BIOCATALYSIS database, our tool offers high-accuracy predictions, reflecting the latest in chemical research and data.
Strategy Settings
Precursor scoring | Relevance Heuristic |
---|---|
Min. plausibility | 0.01 |
Model | Template_relevance |
Template Set | Pistachio/Bkms_metabolic/Pistachio_ringbreaker/Reaxys/Reaxys_biocatalysis |
Top-N result to add to graph | 6 |
Feasible Synthetic Routes
Disclaimer and Information on In-Vitro Research Products
Please be aware that all articles and product information presented on BenchChem are intended solely for informational purposes. The products available for purchase on BenchChem are specifically designed for in-vitro studies, which are conducted outside of living organisms. In-vitro studies, derived from the Latin term "in glass," involve experiments performed in controlled laboratory settings using cells or tissues. It is important to note that these products are not categorized as medicines or drugs, and they have not received approval from the FDA for the prevention, treatment, or cure of any medical condition, ailment, or disease. We must emphasize that any form of bodily introduction of these products into humans or animals is strictly prohibited by law. It is essential to adhere to these guidelines to ensure compliance with legal and ethical standards in research and experimentation.