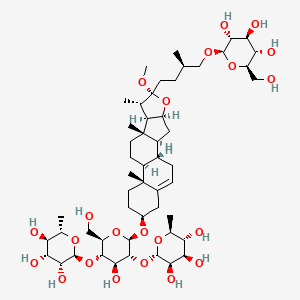
Methylprotodioscin
Overview
Description
Methylprotodioscin is a natural compound found in the rhizomes of Dioscoreaceae, a family of yam plants. It is a furostanol saponin, known for its bioactive properties, including anti-inflammatory and anticancer activities . This compound has been studied for its potential therapeutic effects, particularly in the treatment of various inflammatory conditions and cancers .
Mechanism of Action
Methylprotodioscin, also known as Methyl protodioscin, is a natural furostanol saponin found in the rhizomes of Dioscoreaceae . It has been reported to have lipid-lowering and broad anticancer properties .
Target of Action
This compound primarily targets the Forkhead box O1 (FOXO1) protein and the Mitogen-Activated Protein Kinase (MAPK) signaling pathway . FOXO1 is a tumor suppressor and a critical factor controlling cholesterol metabolism . The MAPK signaling pathway plays a crucial role in cellular proliferation, differentiation, and apoptosis .
Mode of Action
This compound interacts with its targets by inducing the FOXO1 protein and reducing the cholesterol concentration . This disruption of lipid rafts leads to a reduction in the associated MAPK signaling pathway protein P-extracellular regulated protein kinase (ERK) .
Biochemical Pathways
The compound affects the cholesterol-associated MAPK signaling pathway . By reducing cholesterol concentration and disrupting lipid rafts, this compound reduces the MAPK signaling pathway . This results in the suppression of proliferation, migration, invasion, and cell cycle, and induces apoptosis of prostate cancer cells .
Pharmacokinetics
The pharmacokinetics of this compound were investigated in rats after intravenous injection . The plasma concentrations of this compound declined rapidly with an elimination half-life (t1/2) from 25.56 to 29.32 minutes . The compound was found to be distributed and eliminated rapidly in rats .
Result of Action
This compound displays anti-prostate cancer activity by inducing the FOXO1 protein, reducing cholesterol concentration, and disrupting lipid rafts . Consequently, the reduced MAPK signaling pathway suppresses proliferation, migration, invasion, and cell cycle and induces apoptosis of prostate cancer cells .
Biochemical Analysis
Biochemical Properties
Methylprotodioscin plays a crucial role in various biochemical reactions. It interacts with several enzymes, proteins, and other biomolecules, influencing their activity and function. One of the primary interactions of this compound is with the enzyme superoxide dismutase 2, which is involved in the detoxification of reactive oxygen species. This compound has been shown to elevate the levels of superoxide dismutase 2, thereby enhancing the cell’s antioxidant defense mechanism . Additionally, this compound inhibits the enzyme mitochondrial E3 ubiquitin ligase 1, which is involved in the regulation of mitochondrial function and apoptosis . These interactions highlight the compound’s role in modulating oxidative stress and apoptosis pathways.
Cellular Effects
This compound exerts significant effects on various types of cells and cellular processes. In cancer cells, such as pancreatic cancer cells and human lung cancer cells, this compound has been shown to inhibit cell proliferation and induce apoptosis . This compound influences cell signaling pathways, including the p38 mitogen-activated protein kinase pathway, which is involved in the regulation of cell growth and apoptosis . This compound also affects gene expression by upregulating apoptotic-related proteins such as cleaved caspase-3 and cleaved caspase-9, and downregulating anti-apoptotic proteins like Bcl-2 . Furthermore, this compound impacts cellular metabolism by suppressing glycolysis, thereby reducing the energy supply to cancer cells and promoting cell death .
Molecular Mechanism
The molecular mechanism of this compound involves several key interactions at the molecular level. This compound binds to specific biomolecules, such as the proteins NIX and LC3, which are involved in the process of mitophagy . This binding interaction leads to the upregulation of mitophagy-related proteins and the induction of mitochondrial dysfunction, ultimately resulting in apoptosis . Additionally, this compound inhibits the activity of the enzyme p38 mitogen-activated protein kinase, which plays a critical role in cell survival and apoptosis . By modulating these molecular pathways, this compound exerts its anticancer effects and promotes cell death in cancer cells.
Temporal Effects in Laboratory Settings
In laboratory settings, the effects of this compound have been observed to change over time. Studies have shown that this compound is relatively stable under standard laboratory conditions, with minimal degradation over time . The long-term effects of this compound on cellular function have been observed in both in vitro and in vivo studies. In in vitro studies, prolonged exposure to this compound has been shown to result in sustained inhibition of cell proliferation and induction of apoptosis . In in vivo studies, this compound has demonstrated long-term anticancer effects, with significant tumor growth inhibition observed over extended periods .
Dosage Effects in Animal Models
The effects of this compound vary with different dosages in animal models. Studies have shown that low to moderate doses of this compound exhibit significant anticancer effects, with minimal toxicity . At high doses, this compound can induce toxic effects, including hepatotoxicity and nephrotoxicity . Threshold effects have also been observed, where a certain dosage level is required to achieve the desired therapeutic effect. These findings highlight the importance of optimizing the dosage of this compound to maximize its therapeutic benefits while minimizing potential adverse effects.
Metabolic Pathways
This compound is involved in several metabolic pathways, interacting with various enzymes and cofactors. One of the primary metabolic pathways of this compound involves its conversion to protodioscin, a related saponin with similar pharmacological properties . This conversion is mediated by specific enzymes, including glycosidases, which cleave the glycosidic bonds in this compound . Additionally, this compound affects metabolic flux by modulating the activity of key metabolic enzymes, such as those involved in glycolysis and oxidative phosphorylation . These interactions influence the overall metabolic profile of cells and contribute to the compound’s therapeutic effects.
Transport and Distribution
The transport and distribution of this compound within cells and tissues are mediated by various transporters and binding proteins. This compound is known to interact with specific transporters, such as the organic anion-transporting polypeptides, which facilitate its uptake into cells . Once inside the cells, this compound can bind to intracellular proteins, influencing its localization and accumulation . The distribution of this compound within tissues is also influenced by factors such as blood flow and tissue permeability . These factors determine the overall bioavailability and therapeutic efficacy of this compound.
Subcellular Localization
The subcellular localization of this compound plays a crucial role in its activity and function. This compound has been shown to localize primarily to the mitochondria, where it exerts its effects on mitochondrial function and apoptosis . The targeting of this compound to the mitochondria is mediated by specific targeting signals and post-translational modifications . Additionally, this compound can localize to other subcellular compartments, such as the cytosol and nucleus, where it influences various cellular processes . The subcellular localization of this compound is essential for its therapeutic effects and determines its overall efficacy in different cellular contexts.
Preparation Methods
Synthetic Routes and Reaction Conditions
The synthesis of methylprotodioscin involves several steps, starting from the extraction of the compound from natural sources. The process typically includes the hydrolysis of saponins to obtain the aglycone, followed by methylation to produce this compound . Specific reaction conditions, such as temperature, pH, and the use of catalysts, are crucial to ensure the efficiency and yield of the synthesis .
Industrial Production Methods
Industrial production of this compound involves large-scale extraction from Dioscoreaceae plants. The process includes the use of solvents for extraction, followed by purification steps such as chromatography to isolate the compound . Advances in biotechnology have also enabled the production of this compound through microbial fermentation, which offers a more sustainable and scalable approach .
Chemical Reactions Analysis
Types of Reactions
Methylprotodioscin undergoes various chemical reactions, including:
Substitution: This reaction involves the replacement of one functional group with another, often using reagents like halogens or alkylating agents.
Common Reagents and Conditions
Common reagents used in the reactions of this compound include oxidizing agents (e.g., potassium permanganate), reducing agents (e.g., sodium borohydride), and alkylating agents (e.g., methyl iodide) . Reaction conditions such as temperature, pressure, and pH are carefully controlled to optimize the yield and selectivity of the reactions .
Major Products Formed
The major products formed from the reactions of this compound depend on the type of reaction and the reagents used. For example, oxidation reactions may produce hydroxylated derivatives, while reduction reactions may yield deoxygenated compounds . Substitution reactions can result in various alkylated or halogenated derivatives .
Scientific Research Applications
Methylprotodioscin has a wide range of scientific research applications, including:
Comparison with Similar Compounds
Methylprotodioscin is structurally similar to other furostanol saponins, such as protodioscin and dioscin . it is unique in its methylated form, which enhances its bioactivity and stability . Compared to protodioscin, this compound has shown greater efficacy in reducing inflammation and inhibiting cancer cell growth . Other similar compounds include diosgenin and (+)-syringaresinol-O-beta-D-glucoside, which also exhibit bioactive properties but differ in their specific mechanisms of action and therapeutic potential .
Properties
IUPAC Name |
(2S,3R,4R,5R,6S)-2-[(2R,3S,4S,5R,6R)-4-hydroxy-2-(hydroxymethyl)-6-[[(1S,2S,4S,6R,7S,8R,9S,12S,13R,16S)-6-methoxy-7,9,13-trimethyl-6-[(3R)-3-methyl-4-[(2R,3R,4S,5S,6R)-3,4,5-trihydroxy-6-(hydroxymethyl)oxan-2-yl]oxybutyl]-5-oxapentacyclo[10.8.0.02,9.04,8.013,18]icos-18-en-16-yl]oxy]-5-[(2S,3R,4R,5R,6S)-3,4,5-trihydroxy-6-methyloxan-2-yl]oxyoxan-3-yl]oxy-6-methyloxane-3,4,5-triol | |
---|---|---|
Source | PubChem | |
URL | https://pubchem.ncbi.nlm.nih.gov | |
Description | Data deposited in or computed by PubChem | |
InChI |
InChI=1S/C52H86O22/c1-21(20-66-46-40(61)39(60)36(57)31(18-53)70-46)10-15-52(65-7)22(2)33-30(74-52)17-29-27-9-8-25-16-26(11-13-50(25,5)28(27)12-14-51(29,33)6)69-49-45(73-48-42(63)38(59)35(56)24(4)68-48)43(64)44(32(19-54)71-49)72-47-41(62)37(58)34(55)23(3)67-47/h8,21-24,26-49,53-64H,9-20H2,1-7H3/t21-,22+,23+,24+,26+,27-,28+,29+,30+,31-,32-,33+,34+,35+,36-,37-,38-,39+,40-,41-,42-,43+,44-,45-,46-,47+,48+,49-,50+,51+,52-/m1/s1 | |
Source | PubChem | |
URL | https://pubchem.ncbi.nlm.nih.gov | |
Description | Data deposited in or computed by PubChem | |
InChI Key |
HSSJYSJXBOCKQM-GVTGEURHSA-N | |
Source | PubChem | |
URL | https://pubchem.ncbi.nlm.nih.gov | |
Description | Data deposited in or computed by PubChem | |
Canonical SMILES |
CC1C2C(CC3C2(CCC4C3CC=C5C4(CCC(C5)OC6C(C(C(C(O6)CO)OC7C(C(C(C(O7)C)O)O)O)O)OC8C(C(C(C(O8)C)O)O)O)C)C)OC1(CCC(C)COC9C(C(C(C(O9)CO)O)O)O)OC | |
Source | PubChem | |
URL | https://pubchem.ncbi.nlm.nih.gov | |
Description | Data deposited in or computed by PubChem | |
Isomeric SMILES |
C[C@H]1[C@H]2[C@H](C[C@@H]3[C@@]2(CC[C@H]4[C@H]3CC=C5[C@@]4(CC[C@@H](C5)O[C@H]6[C@@H]([C@H]([C@@H]([C@H](O6)CO)O[C@H]7[C@@H]([C@@H]([C@H]([C@@H](O7)C)O)O)O)O)O[C@H]8[C@@H]([C@@H]([C@H]([C@@H](O8)C)O)O)O)C)C)O[C@@]1(CC[C@@H](C)CO[C@H]9[C@@H]([C@H]([C@@H]([C@H](O9)CO)O)O)O)OC | |
Source | PubChem | |
URL | https://pubchem.ncbi.nlm.nih.gov | |
Description | Data deposited in or computed by PubChem | |
Molecular Formula |
C52H86O22 | |
Source | PubChem | |
URL | https://pubchem.ncbi.nlm.nih.gov | |
Description | Data deposited in or computed by PubChem | |
DSSTOX Substance ID |
DTXSID301031695 | |
Record name | Methylprotodioscin | |
Source | EPA DSSTox | |
URL | https://comptox.epa.gov/dashboard/DTXSID301031695 | |
Description | DSSTox provides a high quality public chemistry resource for supporting improved predictive toxicology. | |
Molecular Weight |
1063.2 g/mol | |
Source | PubChem | |
URL | https://pubchem.ncbi.nlm.nih.gov | |
Description | Data deposited in or computed by PubChem | |
CAS No. |
54522-52-0 | |
Record name | Methylprotodioscin | |
Source | CAS Common Chemistry | |
URL | https://commonchemistry.cas.org/detail?cas_rn=54522-52-0 | |
Description | CAS Common Chemistry is an open community resource for accessing chemical information. Nearly 500,000 chemical substances from CAS REGISTRY cover areas of community interest, including common and frequently regulated chemicals, and those relevant to high school and undergraduate chemistry classes. This chemical information, curated by our expert scientists, is provided in alignment with our mission as a division of the American Chemical Society. | |
Explanation | The data from CAS Common Chemistry is provided under a CC-BY-NC 4.0 license, unless otherwise stated. | |
Record name | Methylprotodioscin | |
Source | ChemIDplus | |
URL | https://pubchem.ncbi.nlm.nih.gov/substance/?source=chemidplus&sourceid=0054522520 | |
Description | ChemIDplus is a free, web search system that provides access to the structure and nomenclature authority files used for the identification of chemical substances cited in National Library of Medicine (NLM) databases, including the TOXNET system. | |
Record name | Methylprotodioscin | |
Source | EPA DSSTox | |
URL | https://comptox.epa.gov/dashboard/DTXSID301031695 | |
Description | DSSTox provides a high quality public chemistry resource for supporting improved predictive toxicology. | |
Record name | METHYLPROTODIOSCIN | |
Source | FDA Global Substance Registration System (GSRS) | |
URL | https://gsrs.ncats.nih.gov/ginas/app/beta/substances/1VJB6VV6IA | |
Description | The FDA Global Substance Registration System (GSRS) enables the efficient and accurate exchange of information on what substances are in regulated products. Instead of relying on names, which vary across regulatory domains, countries, and regions, the GSRS knowledge base makes it possible for substances to be defined by standardized, scientific descriptions. | |
Explanation | Unless otherwise noted, the contents of the FDA website (www.fda.gov), both text and graphics, are not copyrighted. They are in the public domain and may be republished, reprinted and otherwise used freely by anyone without the need to obtain permission from FDA. Credit to the U.S. Food and Drug Administration as the source is appreciated but not required. | |
A: Methyl protodioscin (MPD) is a natural furostanol saponin found in plants belonging to the Dioscoreaceae family, such as certain species of yams. [] It has been the subject of extensive research for its diverse pharmacological activities, particularly its anti-tumor, anti-inflammatory, and neuroprotective properties. [, , , , ]
A: MPD has a molecular formula of C51H84O22 and a molecular weight of 1085.2 g/mol. []
ANone: The structure of MPD is elucidated using a combination of spectroscopic techniques, primarily Nuclear Magnetic Resonance (NMR) spectroscopy and Mass Spectrometry (MS). [1H-NMR, 13C-NMR, 1H-1H COSY, HMQC, HMBC, and FAB-MS are commonly employed. 29]
ANone: MPD has been shown to induce apoptosis, or programmed cell death, in various cancer cell lines. Studies suggest that MPD triggers apoptosis via multiple pathways, including:
- Caspase Activation: MPD activates caspase-9 and caspase-3, key enzymes involved in the execution phase of apoptosis. []
- Mitochondrial Pathway: MPD disrupts mitochondrial membrane potential, leading to the release of cytochrome c, a crucial step in the intrinsic apoptotic pathway. []
- MAPK Signaling: MPD modulates the activity of mitogen-activated protein kinases (MAPKs), specifically JNK and p38, which are involved in stress-induced apoptosis. []
- Cell Cycle Arrest: MPD induces cell cycle arrest at the G2/M phase, preventing cancer cells from dividing and proliferating. []
ANone: Yes, in vitro and in vivo studies have demonstrated the anticancer potential of MPD against several cancer types, including:
- Osteosarcoma: MPD inhibits cell growth and induces apoptosis in MG-63 human osteosarcoma cells. []
- Lung Cancer: MPD shows growth inhibitory effects, induces G2/M cell cycle arrest, and triggers apoptosis in A549 human lung cancer cells. []
- Prostate Cancer: MPD suppresses proliferation, migration, invasion, and cell cycle progression while inducing apoptosis in DU145 prostate cancer cells. []
- Pancreatic Cancer: MPD has been found to suppress proliferation and induce apoptosis in pancreatic cancer cells, along with inhibiting glycolysis, a process often upregulated in cancer cells. []
A: MPD has been found to decrease cholesterol concentration, potentially by inducing Forkhead box O (FOXO)1, a tumor suppressor protein involved in cholesterol metabolism. [] This cholesterol-lowering effect may disrupt lipid rafts, specialized membrane microdomains enriched in cholesterol and signaling molecules. The disruption of lipid rafts can negatively impact the function of oncogenic signaling pathways, contributing to MPD's anticancer effects. []
A: Studies suggest that MPD, at therapeutic concentrations, does not significantly inhibit the activity of seven major cytochrome P450 enzymes (CYP1A2, CYP2D6, CYP2C9, CYP2C19, CYP2E1, CYP2A6, and CYP3A4) in human liver microsomes. [] This finding implies a low probability of drug-drug interactions between MPD and drugs metabolized by these enzymes.
A: Pharmacokinetic studies in rats have revealed that MPD exhibits dose-dependent pharmacokinetics following intravenous administration. [, ] The compound is rapidly cleared from the plasma, with an elimination half-life ranging from 25.56 to 29.32 minutes. [] MPD appears to follow a one-compartment model after intravenous injection, indicating rapid distribution and elimination. [] A considerable portion of the administered dose is recovered in urine and bile, suggesting these as primary routes of excretion. []
A: Yes, a highly sensitive and specific high-performance liquid chromatography/tandem mass spectrometry (HPLC-MS/MS) assay has been developed and validated for the quantification of MPD in rat plasma. [] This method utilizes methanol-mediated protein precipitation for sample pretreatment and achieves separation on a C18 column.
A: Yes, the developed HPLC-MS/MS method has been validated for its linearity, specificity, accuracy, precision, and reproducibility, demonstrating its suitability for pharmacokinetic studies. []
ANone: MPD has demonstrated additional pharmacological activities, including:
- Anti-inflammatory effects: MPD reduces airway inflammation by inhibiting the production of pro-inflammatory cytokines like IL-6, IL-8, and TNF-α. []
- Cardioprotective effects: MPD has shown protective effects against myocardial infarction in rats. [] It reduces myocardial enzyme levels, lessens infarct size, and improves the heart's antioxidant capacity. [] It also protects cardiomyocytes from anoxia/reoxygenation injury. []
- Anti-thrombotic effects: MPD displays anti-thrombotic effects in rats by inhibiting both in vitro and in vivo thrombosis. [] It prolongs occlusion time and reduces thrombus weight. [] It also possesses blood viscosity-lowering effects. []
- Anti-osteoporotic activity: Studies have shown that MPD and extracts rich in MPD can inhibit bone loss in ovariectomized rats and mice, indicating potential in treating osteoporosis. []
A: Modifications to the MPD structure can significantly impact its biological activity. For example, the introduction of specific sugar moieties or acyl groups can enhance its anti-inflammatory properties. [] Further research is ongoing to fully understand the SAR of MPD and develop more potent and selective analogs.
A: Yes, several synthetic routes to MPD have been reported. These typically involve multi-step procedures starting from diosgenin, a related steroidal sapogenin. [, , ] Improvements in synthetic strategies have led to more efficient and scalable methods for obtaining MPD and its analogs.
ANone: Despite its promising pharmacological activities, there are challenges in translating MPD into a clinically viable drug. These include:
- Low water solubility: MPD's poor water solubility can hinder its formulation and bioavailability. []
ANone: Yes, researchers are exploring various strategies to enhance the therapeutic profile of MPD, such as:
A: The presence and distribution of MPD and related saponins within specific plant families, like Dioscoreaceae and Palmae, provide valuable insights into plant evolution and classification. [] Chemotaxonomic studies based on MPD can contribute to a better understanding of plant biodiversity and the discovery of new bioactive compounds.
A: Xanthine oxidase is an enzyme involved in purine metabolism. Its inhibition can lead to a decrease in uric acid production, making MPD a potential therapeutic candidate for hyperuricemia and gout. [, ]
A: Computational chemistry plays a crucial role in understanding the interactions between MPD and its biological targets, predicting the properties of new analogs, and guiding the design of improved therapeutics. Techniques like molecular docking and molecular dynamics simulations can provide valuable insights into the binding modes, affinities, and potential mechanisms of action of MPD and its derivatives. []
Disclaimer and Information on In-Vitro Research Products
Please be aware that all articles and product information presented on BenchChem are intended solely for informational purposes. The products available for purchase on BenchChem are specifically designed for in-vitro studies, which are conducted outside of living organisms. In-vitro studies, derived from the Latin term "in glass," involve experiments performed in controlled laboratory settings using cells or tissues. It is important to note that these products are not categorized as medicines or drugs, and they have not received approval from the FDA for the prevention, treatment, or cure of any medical condition, ailment, or disease. We must emphasize that any form of bodily introduction of these products into humans or animals is strictly prohibited by law. It is essential to adhere to these guidelines to ensure compliance with legal and ethical standards in research and experimentation.