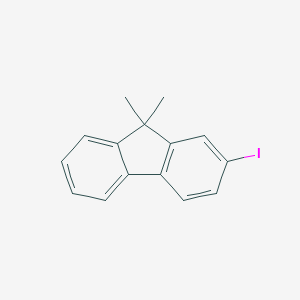
2-iodo-9,9-dimethyl-9H-fluorene
Overview
Description
2-Iodo-9,9-dimethyl-9H-fluorene is an organic compound with the chemical formula C15H13I. It is an organic halide and appears as a white to pale yellow crystalline substance . This compound is primarily used in organic synthesis, particularly as a starting material for the synthesis of aromatic compounds .
Mechanism of Action
Pharmacokinetics
It is a substrate for P-gp and an inhibitor for CYP1A2 and CYP2C19 . Its lipophilicity (Log Po/w) is 3.04 (iLOGP) and 5.07 (XLOGP3) .
Action Environment
The action of 2-Iodo-9,9-dimethyl-9H-fluorene can be influenced by environmental factors such as temperature and light. It is recommended to be stored in a dark place, sealed in dry conditions, at room temperature . These conditions likely help maintain the stability and efficacy of the compound.
Preparation Methods
The preparation of 2-iodo-9,9-dimethyl-9H-fluorene can be achieved through the iodination of 2-fluorenyl-9,9-dimethylfluorene. A common method involves reacting 2-fluorenyl-9,9-dimethylfluorene with iodide in a suitable solvent . The reaction conditions typically include maintaining the reaction mixture at a specific temperature and using a catalyst to facilitate the iodination process .
Chemical Reactions Analysis
2-Iodo-9,9-dimethyl-9H-fluorene undergoes various types of chemical reactions, including:
Substitution Reactions: This compound can participate in nucleophilic substitution reactions where the iodine atom is replaced by other nucleophiles.
Oxidation Reactions: It can be oxidized to form different products depending on the reagents and conditions used.
Reduction Reactions: Reduction of this compound can lead to the formation of 9,9-dimethylfluorene.
Common reagents used in these reactions include halides, oxidizing agents, and reducing agents. The major products formed from these reactions vary based on the specific conditions and reagents employed .
Scientific Research Applications
2-Iodo-9,9-dimethyl-9H-fluorene has several scientific research applications:
Organic Synthesis: It is used as a starting material for synthesizing various aromatic compounds, including fluorescent dyes, drugs, and polymer materials.
Material Science: This compound is utilized in the development of organic electronic materials, such as organic light-emitting diodes (OLEDs) and organic solar cells (OSCs).
Biological Research: It can be used in the synthesis of biologically active molecules for medicinal chemistry research.
Comparison with Similar Compounds
2-Iodo-9,9-dimethyl-9H-fluorene can be compared with other similar compounds, such as:
2-Bromo-9,9-dimethylfluorene: This compound has a bromine atom instead of an iodine atom, which affects its reactivity and applications.
2,7-Diiodo-9,9-dimethyl-9H-fluorene:
2-Iodo-9H-fluorene: This compound lacks the dimethyl groups, which can influence its chemical properties and uses.
The uniqueness of this compound lies in its specific structure, which provides distinct reactivity and applications in various fields of research and industry .
Properties
IUPAC Name |
2-iodo-9,9-dimethylfluorene | |
---|---|---|
Source | PubChem | |
URL | https://pubchem.ncbi.nlm.nih.gov | |
Description | Data deposited in or computed by PubChem | |
InChI |
InChI=1S/C15H13I/c1-15(2)13-6-4-3-5-11(13)12-8-7-10(16)9-14(12)15/h3-9H,1-2H3 | |
Source | PubChem | |
URL | https://pubchem.ncbi.nlm.nih.gov | |
Description | Data deposited in or computed by PubChem | |
InChI Key |
DVLSJPCXPNKPRJ-UHFFFAOYSA-N | |
Source | PubChem | |
URL | https://pubchem.ncbi.nlm.nih.gov | |
Description | Data deposited in or computed by PubChem | |
Canonical SMILES |
CC1(C2=CC=CC=C2C3=C1C=C(C=C3)I)C | |
Source | PubChem | |
URL | https://pubchem.ncbi.nlm.nih.gov | |
Description | Data deposited in or computed by PubChem | |
Molecular Formula |
C15H13I | |
Source | PubChem | |
URL | https://pubchem.ncbi.nlm.nih.gov | |
Description | Data deposited in or computed by PubChem | |
DSSTOX Substance ID |
DTXSID80452321 | |
Record name | 2-iodo-9,9-dimethyl-9H-fluorene | |
Source | EPA DSSTox | |
URL | https://comptox.epa.gov/dashboard/DTXSID80452321 | |
Description | DSSTox provides a high quality public chemistry resource for supporting improved predictive toxicology. | |
Molecular Weight |
320.17 g/mol | |
Source | PubChem | |
URL | https://pubchem.ncbi.nlm.nih.gov | |
Description | Data deposited in or computed by PubChem | |
CAS No. |
144981-85-1 | |
Record name | 2-iodo-9,9-dimethyl-9H-fluorene | |
Source | EPA DSSTox | |
URL | https://comptox.epa.gov/dashboard/DTXSID80452321 | |
Description | DSSTox provides a high quality public chemistry resource for supporting improved predictive toxicology. | |
Synthesis routes and methods I
Procedure details
Synthesis routes and methods II
Procedure details
Retrosynthesis Analysis
AI-Powered Synthesis Planning: Our tool employs the Template_relevance Pistachio, Template_relevance Bkms_metabolic, Template_relevance Pistachio_ringbreaker, Template_relevance Reaxys, Template_relevance Reaxys_biocatalysis model, leveraging a vast database of chemical reactions to predict feasible synthetic routes.
One-Step Synthesis Focus: Specifically designed for one-step synthesis, it provides concise and direct routes for your target compounds, streamlining the synthesis process.
Accurate Predictions: Utilizing the extensive PISTACHIO, BKMS_METABOLIC, PISTACHIO_RINGBREAKER, REAXYS, REAXYS_BIOCATALYSIS database, our tool offers high-accuracy predictions, reflecting the latest in chemical research and data.
Strategy Settings
Precursor scoring | Relevance Heuristic |
---|---|
Min. plausibility | 0.01 |
Model | Template_relevance |
Template Set | Pistachio/Bkms_metabolic/Pistachio_ringbreaker/Reaxys/Reaxys_biocatalysis |
Top-N result to add to graph | 6 |
Feasible Synthetic Routes
Disclaimer and Information on In-Vitro Research Products
Please be aware that all articles and product information presented on BenchChem are intended solely for informational purposes. The products available for purchase on BenchChem are specifically designed for in-vitro studies, which are conducted outside of living organisms. In-vitro studies, derived from the Latin term "in glass," involve experiments performed in controlled laboratory settings using cells or tissues. It is important to note that these products are not categorized as medicines or drugs, and they have not received approval from the FDA for the prevention, treatment, or cure of any medical condition, ailment, or disease. We must emphasize that any form of bodily introduction of these products into humans or animals is strictly prohibited by law. It is essential to adhere to these guidelines to ensure compliance with legal and ethical standards in research and experimentation.