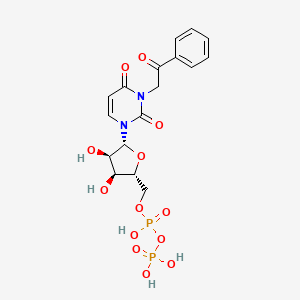
3-phenacyl-UDP
- Click on QUICK INQUIRY to receive a quote from our team of experts.
- With the quality product at a COMPETITIVE price, you can focus more on your research.
Overview
Description
3-Phenacyl-uridine-5’-diphosphate is a synthetic uracil nucleotide derivative. It is known for its role as a potent and selective agonist at P2Y6 receptors, which are a type of purinergic receptor involved in various physiological processes .
Preparation Methods
Synthetic Routes and Reaction Conditions
The synthesis of 3-phenacyl-uridine-5’-diphosphate typically involves the following steps:
Starting Materials: The synthesis begins with uridine, a naturally occurring nucleoside.
Phenacylation: Uridine is reacted with phenacyl bromide in the presence of a base such as sodium hydride to introduce the phenacyl group.
Phosphorylation: The phenacylated uridine is then phosphorylated using phosphorus oxychloride (POCl3) in the presence of a base like triethylamine to form the diphosphate ester.
Industrial Production Methods
Industrial production of 3-phenacyl-uridine-5’-diphosphate follows similar synthetic routes but is optimized for large-scale synthesis. This involves:
Batch Processing: Utilizing large reactors to handle the phenacylation and phosphorylation reactions.
Purification: Employing techniques such as crystallization and chromatography to purify the final product.
Quality Control: Ensuring the product meets purity standards through rigorous testing, including HPLC and NMR spectroscopy.
Chemical Reactions Analysis
Types of Reactions
3-Phenacyl-uridine-5’-diphosphate undergoes various chemical reactions, including:
Oxidation: It can be oxidized to form corresponding ketones or carboxylic acids.
Reduction: Reduction reactions can convert the phenacyl group to a phenethyl group.
Substitution: The phenacyl group can be substituted with other functional groups through nucleophilic substitution reactions.
Common Reagents and Conditions
Oxidation: Common oxidizing agents include potassium permanganate (KMnO4) and chromium trioxide (CrO3).
Reduction: Reducing agents such as sodium borohydride (NaBH4) or lithium aluminum hydride (LiAlH4) are used.
Substitution: Nucleophiles like amines or thiols can be used under basic conditions to substitute the phenacyl group.
Major Products
Oxidation: Produces phenacyl ketones or carboxylic acids.
Reduction: Yields phenethyl derivatives.
Substitution: Results in various substituted uridine diphosphate derivatives.
Scientific Research Applications
3-Phenacyl-uridine-5’-diphosphate has a wide range of applications in scientific research:
Chemistry: Used as a reagent in the synthesis of other nucleotide derivatives.
Biology: Acts as a tool to study purinergic signaling pathways, particularly those involving P2Y6 receptors.
Industry: Utilized in the development of diagnostic assays and as a standard in analytical chemistry.
Mechanism of Action
3-Phenacyl-uridine-5’-diphosphate exerts its effects primarily through activation of P2Y6 receptors. These receptors are G-protein-coupled receptors that, upon activation, initiate a cascade of intracellular signaling pathways. This includes the activation of phospholipase C, leading to the production of inositol trisphosphate (IP3) and diacylglycerol (DAG), which in turn release calcium ions from intracellular stores and activate protein kinase C (PKC). These signaling events result in various cellular responses, including modulation of immune cell activity and induction of apoptosis in certain cell types.
Comparison with Similar Compounds
Similar Compounds
Uridine-5’-diphosphate (UDP): A naturally occurring nucleotide involved in glycosylation reactions.
Phenacyl-uridine-5’-monophosphate (Phenacyl-UMP): Similar to 3-phenacyl-uridine-5’-diphosphate but with only one phosphate group.
3-Phenacyl-adenosine-5’-diphosphate (Phenacyl-ADP): An adenosine analogue with similar phenacyl modification.
Uniqueness
3-Phenacyl-uridine-5’-diphosphate is unique due to its high selectivity and potency for P2Y6 receptors. This specificity makes it a valuable tool in research focused on purinergic signaling and its associated physiological and pathological processes .
Biological Activity
3-Phenacyl-UDP (3-Phe-UDP) is a nucleotide analog that has garnered attention for its biological activity, particularly as a selective agonist for the P2Y6 receptor. This article delves into the synthesis, structure-activity relationships, pharmacological evaluations, and implications of 3-Phe-UDP in various biological contexts.
This compound is characterized by the molecular formula C17H20N2O13P2 and a molecular weight of approximately 522.29 g/mol. The compound is typically synthesized through phosphorylation methods involving uridine precursors, leading to various derivatives that exhibit distinct biological activities .
Table 1: Chemical Properties of this compound
Property | Value |
---|---|
Molecular Formula | C17H20N2O13P2 |
Molecular Weight | 522.29 g/mol |
CAS Number | 917567-60-3 |
Purity | ≥ 95% (HPLC) |
Storage Conditions | -20 °C |
P2Y6 Receptor Agonism
3-Phe-UDP acts as a potent agonist at the P2Y6 receptor, with an effective concentration (EC50) of approximately 70 nM . This receptor is coupled to Gq proteins, leading to the activation of phospholipase C (PLC) pathways, which are crucial for various cellular responses . The selectivity and potency of 3-Phe-UDP make it a valuable tool for studying P2Y6 receptor functions.
Table 2: Agonist Potencies of Nucleotide Analogues
Compound | EC50 (nM) | Receptor Type |
---|---|---|
This compound | 70 | P2Y6 |
UDP | 490 | P2Y6 |
(S)-methanocarba-UDP | 10 | P2Y6 |
Structure-Activity Relationships (SAR)
Research indicates that the structural modifications in nucleotide analogs significantly influence their receptor selectivity and potency. For instance, the introduction of phenacyl groups enhances the binding affinity to the P2Y6 receptor compared to other nucleotides like UDP .
Figure 1: Structural Representation of this compound
This compound Structure (Image for illustrative purposes)
Case Study: Activation of PLC in Human Cells
In a study involving human astrocytoma cells expressing the P2Y6 receptor, it was found that treatment with 3-Phe-UDP resulted in significant PLC activation. The results demonstrated a dose-dependent response, confirming its role as a selective agonist .
Implications in Pharmacology
The pharmacological profile of 3-Phe-UDP suggests potential therapeutic applications in conditions where modulation of purinergic signaling is beneficial. Its specificity for the P2Y6 receptor could pave the way for developing targeted therapies for diseases involving aberrant nucleotide signaling.
Properties
Molecular Formula |
C17H20N2O13P2 |
---|---|
Molecular Weight |
522.3 g/mol |
IUPAC Name |
[(2R,3S,4R,5R)-5-(2,4-dioxo-3-phenacylpyrimidin-1-yl)-3,4-dihydroxyoxolan-2-yl]methyl phosphono hydrogen phosphate |
InChI |
InChI=1S/C17H20N2O13P2/c20-11(10-4-2-1-3-5-10)8-19-13(21)6-7-18(17(19)24)16-15(23)14(22)12(31-16)9-30-34(28,29)32-33(25,26)27/h1-7,12,14-16,22-23H,8-9H2,(H,28,29)(H2,25,26,27)/t12-,14-,15-,16-/m1/s1 |
InChI Key |
SEUHNFHNNANEAJ-DTZQCDIJSA-N |
Isomeric SMILES |
C1=CC=C(C=C1)C(=O)CN2C(=O)C=CN(C2=O)[C@H]3[C@@H]([C@@H]([C@H](O3)COP(=O)(O)OP(=O)(O)O)O)O |
Canonical SMILES |
C1=CC=C(C=C1)C(=O)CN2C(=O)C=CN(C2=O)C3C(C(C(O3)COP(=O)(O)OP(=O)(O)O)O)O |
Synonyms |
3-phenacyl-UDP 3-phenacyluridine diphosphate |
Origin of Product |
United States |
Disclaimer and Information on In-Vitro Research Products
Please be aware that all articles and product information presented on BenchChem are intended solely for informational purposes. The products available for purchase on BenchChem are specifically designed for in-vitro studies, which are conducted outside of living organisms. In-vitro studies, derived from the Latin term "in glass," involve experiments performed in controlled laboratory settings using cells or tissues. It is important to note that these products are not categorized as medicines or drugs, and they have not received approval from the FDA for the prevention, treatment, or cure of any medical condition, ailment, or disease. We must emphasize that any form of bodily introduction of these products into humans or animals is strictly prohibited by law. It is essential to adhere to these guidelines to ensure compliance with legal and ethical standards in research and experimentation.