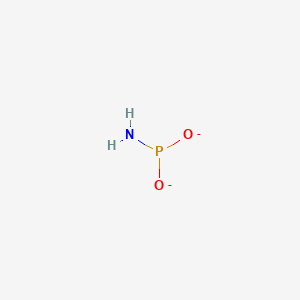
Phosphoramidite
Overview
Description
Phosphoramidites are organophosphorus compounds characterized by a trivalent phosphorus center bonded to an amine group, two alkoxy groups, and a reactive site (typically a hydroxyl-protected nucleoside or other functional group). They are pivotal in solid-phase oligonucleotide synthesis, enabling the stepwise assembly of DNA and RNA strands via the phosphoramidite method . Their structure (P(III)) allows for controlled activation under mild acidic conditions, forming phosphite triester intermediates that oxidize to stable phosphate linkages . Phosphoramidites are widely used in biotechnology, drug development, and molecular diagnostics due to their compatibility with automated synthesis and high coupling efficiency .
Scientific Research Applications
Key Applications
-
Oligonucleotide Synthesis
Phosphoramidites are extensively used to synthesize oligonucleotides for various applications including:- Gene Expression Studies : Modified oligonucleotides can be employed as molecular probes to study protein-nucleic acid interactions, which are fundamental in understanding cellular processes .
- Therapeutic Agents : Antisense oligonucleotides designed from phosphoramidites can inhibit gene expression by binding to target mRNA sequences, offering potential treatments for genetic disorders .
-
Modified Oligonucleotides
Recent advancements have led to the development of modified phosphoramidites that enhance the properties of synthesized oligonucleotides:- Nuclease Resistance : Modifications to phosphodiester linkages improve the stability of oligonucleotides against enzymatic degradation, making them suitable for therapeutic applications .
- Increased Membrane Permeability : Alterations in sugar or backbone units facilitate better cellular uptake of oligonucleotides, enhancing their effectiveness as drugs .
-
Thermal Stability Studies
The thermal stability of phosphoramidites is critical for safe and effective synthesis. Recent studies have shown significant variations in thermal stability among different phosphoramidites, impacting their use in oligonucleotide synthesis . Understanding these properties helps optimize synthesis conditions and improve safety protocols. -
Innovative Synthesis Techniques
Advances in synthetic methodologies, such as on-demand flow synthesis of phosphoramidites, allow for rapid and efficient production of these compounds . This innovation supports high-throughput applications in research and drug development.
Case Study 1: Antisense Oligonucleotides
A study demonstrated the efficacy of antisense oligonucleotides synthesized from modified phosphoramidites in blocking gene expression. These oligonucleotides showed improved binding affinity and specificity towards target mRNA sequences, leading to significant reductions in target gene expression levels .
Case Study 2: siRNA Development
Research on 2′-formamidonucleoside phosphoramidites illustrated their incorporation into small interfering RNA (siRNA) strands. This modification reduced off-target effects while maintaining structural integrity, showcasing the potential for targeted gene silencing therapies .
Data Tables
Chemical Reactions Analysis
Step 1: Detritylation
-
Reaction : Removal of the 5′-dimethoxytrityl (DMT) protecting group using trichloroacetic acid (TCA) in dichloromethane.
-
Mechanism : Acidic cleavage generates a DMT carbocation (detectable at 495 nm) and exposes the 5′-OH for subsequent coupling .
-
Critical Parameters :
Step 2: Coupling
-
Activation : this compound monomers (e.g., 2-cyanoethyl-N,N-diisopropylthis compound) are activated by acidic catalysts like 1H-tetrazole derivatives (e.g., ETT, DCI) .
-
Mechanism :
Step 3: Oxidation
-
Reagents : Iodine/water/pyridine oxidizes the phosphite triester to a phosphate triester .
-
Side Reaction Mitigation : Stabilizes the backbone against hydrolysis during subsequent cycles .
Step 4: Capping
-
Purpose : Acetylation (e.g., acetic anhydride/N-methylimidazole) of unreacted 5′-OH groups prevents deletion errors .
Activation Mechanisms and Kinetics
This compound reactivity is governed by activation pathways:
Activator | Reaction Rate (k, M⁻¹s⁻¹) | Conditions | Source |
---|---|---|---|
1H-Tetrazole | 0.12 | Anhydrous CH₃CN, 25°C | |
Saccharin/NMI complex | 1.8 | CH₂Cl₂, 25°C | |
DCI | 0.45 | CH₃CN, 25°C |
Key Findings :
-
Saccharin-based activators accelerate coupling 15-fold compared to tetrazole .
-
Steric hindrance in nucleosides (e.g., 2′-O-methyl modifications) reduces coupling efficiency by 30–50% .
Deprotection Chemistry
Post-synthesis processing removes protecting groups:
Base Deprotection
-
Protecting Groups :
Phosphate Deprotection
Side Reactions and Error Rates
Common synthetic errors and their prevalence:
Q & A
Basic Research Questions
Q. What is the role of phosphoramidites in oligonucleotide synthesis, and how does their chemical structure influence coupling efficiency?
Phosphoramidites are nucleotide derivatives activated for solid-phase synthesis. Their structure includes a reactive trivalent phosphorus atom, enabling sequential coupling to a growing oligonucleotide chain. The 5'-OH group is protected by a dimethoxytrityl (DMT) group, while the 3'-phosphite is activated for nucleophilic attack. Coupling efficiency depends on the steric hindrance of protecting groups (e.g., benzoyl for adenine, isobutyryl for guanine) and solvent polarity. Acetonitrile (MeCN) is preferred over tetrahydrofuran (THF) due to higher coupling rates and reduced side reactions .
Q. What purification methods are critical for removing truncated sequences or byproducts in phosphoramidite-based oligonucleotide synthesis?
Reverse-phase HPLC is essential for separating full-length oligonucleotides from failure sequences. Key steps include:
- Deprotection : Use ammonium hydroxide to cleave base-labile protecting groups.
- Desalting : Remove salts and small molecules via size-exclusion chromatography.
- HPLC parameters : Utilize C18 columns with gradients of acetonitrile/water (0.1 M triethylammonium acetate buffer) to resolve products by hydrophobicity .
Q. How do oxidation and capping steps minimize deletion errors during this compound synthesis?
- Oxidation : Converts the phosphite triester intermediate to a stable phosphate triester using iodine/water/pyridine.
- Capping : Acetic anhydride and 1-methylimidazole block unreacted 5'-OH groups, preventing further elongation of failure sequences. These steps reduce truncated sequences by >95% when optimized .
Advanced Research Questions
Q. How can solvent-induced peroxide formation degrade phosphoramidites, and what mitigation strategies are effective?
Ethers like THF accumulate peroxides over time, oxidizing phosphoramidites and reducing coupling efficiency. For example, THF peroxide decreases this compound purity by 15–20% within 24 hours, leading to shortmer accumulation. Solutions :
- Use fresh, inhibitor-stabilized THF or replace with MeCN.
- Test solvents for peroxides using potassium iodide/starch strips before synthesis.
- Add antioxidants like butylated hydroxytoluene (BHT) at 0.1% w/v .
Q. What analytical techniques resolve contradictions in this compound reactivity data across literature studies?
Discrepancies arise from variations in solvent purity, activator choice (e.g., tetrazole vs. 5-ethylthio-1H-tetrazole), and humidity control. A standardized protocol includes:
- Kinetic analysis : Measure coupling rates via UV monitoring of DMT cleavage.
- Mass spectrometry : Verify this compound integrity pre- and post-synthesis.
- Controlled environment : Use anhydrous solvents (<10 ppm H₂O) and argon-purged reaction chambers .
Q. How do fluorophore-labeled phosphoramidites (e.g., R6G or Yakima Yellow) impact probe specificity and background noise in fluorescence-based assays?
Fluorophores like R6G (λₑₓ = 518 nm, λₑₘ = 542 nm) require precise positioning to avoid steric interference. For example:
- Eclipse probes : Place quenchers (e.g., MGB) at the 3'-end and fluorophores at the 5'-end to minimize background.
- Purification : HPLC removes unincorporated dyes, reducing false signals by 90% .
Q. Methodological Recommendations
- Synthetic Optimization : Pre-dry phosphoramidites and solvents over 3Å molecular sieves for 16 hours to minimize hydrolysis .
- Quality Control : Use MALDI-TOF MS to confirm oligonucleotide mass and UV-Vis spectroscopy to quantify dye incorporation .
- Troubleshooting : If coupling efficiency drops below 90%, replace tetrazole activators and verify solvent peroxide levels .
Comparison with Similar Compounds
Phosphoramidates
Phosphoramidates contain a pentavalent phosphorus atom bonded to an amine and three oxygen atoms. Phosphoramidates are utilized in prodrug strategies (e.g., antiviral agents) due to their enzymatic hydrolysis into active metabolites . For example, TriPPPro-d4TTPs, synthesized via the phosphoramidite route, incorporate biodegradable masking groups for targeted drug delivery . However, their synthesis often yields lower efficiency (26–70%) compared to phosphoramidites .
DiPPro-Prodrugs
DiPPro-prodrugs employ this compound chemistry but react with d4TMP (deoxythymidine monophosphate) instead of d4TDP. This modification reduces steric hindrance, improving reaction kinetics and yields compared to TriPPPro-d4TTPs . Both classes share similar P(III) activation mechanisms but differ in nucleotide substrates and applications (e.g., DiPPro-prodrugs focus on nucleotide analog delivery) .
Fluorinated Phosphoramidites
Fluorinated phosphoramidites, such as DMT-2′-Fluoro-dU this compound, incorporate fluorine atoms to enhance oligonucleotide stability and nuclease resistance. These modifications are critical for antisense therapies and CRISPR guide RNAs . Fluorination alters electronic properties, slowing coupling rates compared to non-fluorinated analogs but improving final product purity (98% vs. 90–95% for standard amidites) .
Phosphonates
Phosphonates feature a direct carbon-phosphorus bond, conferring metabolic stability. Their synthesis avoids oxidation steps but requires harsh conditions (e.g., Michaelis-Arbuzov reactions), limiting compatibility with sensitive substrates .
Data Tables
Table 1: Structural and Functional Comparison
Compound | Phosphorus Valency | Key Functional Groups | Primary Use | Stability |
---|---|---|---|---|
This compound | P(III) | Amine, alkoxy, nucleoside | Oligonucleotide synthesis | Air-sensitive |
Phosphoramidate | P(V) | Amine, three oxygens | Prodrugs, antivirals | High |
DiPPro-Prodrug | P(III) | Amine, alkoxy, d4TMP | Nucleotide analog delivery | Moderate |
Fluorinated Amidite | P(III) | Amine, alkoxy, fluorine | Modified oligonucleotides | Moderate |
Research Findings
- Stability : Phosphoramidites degrade in THF due to peroxide formation, reducing coupling efficiency by 20–30% compared to acetonitrile .
- Reactivity : Activation with 1H-tetrazole in acetonitrile achieves >95% this compound conversion in 15–30 minutes, while fluorinated analogs require extended times (30–45 minutes) .
- Applications : Modified phosphoramidites (e.g., 2′-fluoro) enable synthesis of base-labile oligonucleotides for mRNA vaccines, withstanding harsh deprotection conditions .
Properties
Molecular Formula |
H2NO2P-2 |
---|---|
Molecular Weight |
78.995 g/mol |
InChI |
InChI=1S/H2NO2P/c1-4(2)3/h1H2/q-2 |
InChI Key |
LLKYUHGUYSLMPA-UHFFFAOYSA-N |
Canonical SMILES |
NP([O-])[O-] |
Synonyms |
phosphoramidite |
Origin of Product |
United States |
Synthesis routes and methods
Procedure details
Disclaimer and Information on In-Vitro Research Products
Please be aware that all articles and product information presented on BenchChem are intended solely for informational purposes. The products available for purchase on BenchChem are specifically designed for in-vitro studies, which are conducted outside of living organisms. In-vitro studies, derived from the Latin term "in glass," involve experiments performed in controlled laboratory settings using cells or tissues. It is important to note that these products are not categorized as medicines or drugs, and they have not received approval from the FDA for the prevention, treatment, or cure of any medical condition, ailment, or disease. We must emphasize that any form of bodily introduction of these products into humans or animals is strictly prohibited by law. It is essential to adhere to these guidelines to ensure compliance with legal and ethical standards in research and experimentation.