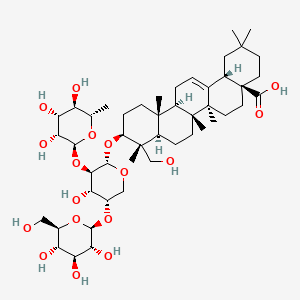
Pulsatilla saponin D
Overview
Description
Scientific Research Applications
Pulsatilla saponin D has a wide range of scientific research applications:
Mechanism of Action
Target of Action
Pulsatilla Saponin D (PSD), isolated from the root of Pulsatilla koreana, primarily targets c-Met , a receptor tyrosine kinase . The c-Met receptor plays a crucial role in various cellular processes, including cell growth, survival, and migration .
Mode of Action
PSD interacts with its target, c-Met, and inhibits its activation . This inhibition of c-Met leads to a decrease in the activation of downstream signaling pathways, including the Akt and mammalian target of rapamycin (mTOR) pathways . The inhibition of these pathways by PSD is dose-dependent .
Biochemical Pathways
The primary biochemical pathways affected by PSD are those downstream of the c-Met receptor. PSD inhibits the activation of the Akt and mTOR pathways, which are involved in cell growth and survival . By inhibiting these pathways, PSD can effectively inhibit the growth of cancer cells .
Pharmacokinetics
It’s known that natural saponins, like psd, have different degrees of lipophilic properties and sugar chains have strong hydrophilic properties . This could potentially influence the bioavailability of PSD, but more research is needed to fully understand these properties.
Result of Action
The inhibition of c-Met and its downstream pathways by PSD leads to a variety of cellular effects. These include the inhibition of gastric cancer cell growth, the induction of apoptosis (programmed cell death), and the inhibition of angiogenesis (the formation of new blood vessels from pre-existing vessels) . These effects contribute to the overall anti-cancer activity of PSD .
Safety and Hazards
Future Directions
Biochemical Analysis
Biochemical Properties
Pulsatilla saponin D plays a crucial role in various biochemical reactions. It interacts with several enzymes, proteins, and other biomolecules, influencing their activity and function. For instance, this compound has been shown to inhibit the activity of matrix metalloproteinases, which are enzymes involved in the degradation of extracellular matrix components . Additionally, it interacts with proteins involved in cell signaling pathways, such as the mitogen-activated protein kinase (MAPK) pathway, modulating their activity and downstream effects . These interactions highlight the compound’s potential in regulating cellular processes and maintaining homeostasis.
Cellular Effects
This compound exerts significant effects on various types of cells and cellular processes. It has been reported to induce apoptosis in cancer cells by activating caspase-independent cell death pathways . Furthermore, this compound influences cell signaling pathways, such as the phosphoinositide 3-kinase (PI3K)/Akt pathway, leading to alterations in gene expression and cellular metabolism . These effects contribute to the compound’s ability to inhibit tumor growth and promote cell death in cancerous cells.
Molecular Mechanism
The molecular mechanism of this compound involves its binding interactions with specific biomolecules, enzyme inhibition or activation, and changes in gene expression. This compound has been shown to bind to the c-Met receptor, inhibiting its activity and downstream signaling pathways . This inhibition leads to reduced angiogenesis and tumor growth. Additionally, this compound modulates the expression of genes involved in apoptosis and cell cycle regulation, further contributing to its anticancer effects .
Temporal Effects in Laboratory Settings
In laboratory settings, the effects of this compound have been observed to change over time. The compound exhibits stability under various conditions, maintaining its bioactivity for extended periods . Degradation of this compound can occur under certain conditions, leading to a reduction in its efficacy . Long-term studies have shown that this compound can induce sustained changes in cellular function, including prolonged inhibition of tumor growth and modulation of immune responses .
Dosage Effects in Animal Models
The effects of this compound vary with different dosages in animal models. At low doses, the compound exhibits minimal toxicity and exerts beneficial effects, such as anti-inflammatory and anticancer activities . At high doses, this compound can induce toxic effects, including hepatotoxicity and nephrotoxicity . Threshold effects have been observed, where the compound’s efficacy plateaus at certain concentrations, indicating the importance of optimizing dosage for therapeutic applications .
Metabolic Pathways
This compound is involved in various metabolic pathways, interacting with enzymes and cofactors that regulate its metabolism. The compound is metabolized by cytochrome P450 enzymes, leading to the formation of active metabolites . These metabolites can further interact with other biomolecules, influencing metabolic flux and metabolite levels . This compound also affects the expression of genes involved in metabolic pathways, contributing to its overall bioactivity .
Transport and Distribution
Within cells and tissues, this compound is transported and distributed through interactions with specific transporters and binding proteins. The compound is known to interact with ATP-binding cassette (ABC) transporters, facilitating its efflux from cells . Additionally, this compound can bind to serum proteins, influencing its distribution and accumulation in various tissues . These interactions play a crucial role in determining the compound’s bioavailability and therapeutic potential .
Subcellular Localization
This compound exhibits specific subcellular localization, which affects its activity and function. The compound is primarily localized in the cytoplasm and can be directed to specific compartments or organelles through targeting signals and post-translational modifications . This localization is essential for its interaction with intracellular biomolecules and the modulation of cellular processes . This compound’s subcellular distribution contributes to its overall bioactivity and therapeutic potential .
Preparation Methods
Synthetic Routes and Reaction Conditions
Pulsatilla saponin D can be isolated from the roots of Pulsatilla chinensis and Pulsatilla koreana using solvent extraction methods . The process involves the use of solvents such as methanol or ethanol to extract the saponins, followed by purification using chromatographic techniques .
Industrial Production Methods
In industrial settings, the extraction of this compound is scaled up using large-scale solvent extraction and purification processes. The roots of Pulsatilla species are processed in bulk, and the saponins are extracted using industrial-grade solvents. The crude extract is then subjected to various purification steps, including column chromatography and crystallization, to obtain pure this compound .
Chemical Reactions Analysis
Types of Reactions
Pulsatilla saponin D undergoes various chemical reactions, including:
Oxidation: this compound can be oxidized to form different derivatives.
Reduction: Reduction reactions can modify the functional groups in this compound.
Substitution: Substitution reactions can introduce new functional groups into the molecule.
Common Reagents and Conditions
Oxidation: Common oxidizing agents include potassium permanganate and hydrogen peroxide.
Reduction: Reducing agents such as sodium borohydride and lithium aluminum hydride are used.
Substitution: Reagents like alkyl halides and acyl chlorides are used in substitution reactions.
Major Products
The major products formed from these reactions include various derivatives of this compound with modified functional groups, which can exhibit different pharmacological activities .
Comparison with Similar Compounds
Similar Compounds
Pulchinenoside A: Another triterpenoid saponin with anticancer and anti-inflammatory properties.
Pulchinenoside C: Known for its antimicrobial and anticancer activities.
Pulsatilloside E: Exhibits similar pharmacological activities, including anticancer and anti-inflammatory effects.
Uniqueness
Pulsatilla saponin D is unique due to its potent anticancer activity and its ability to selectively induce apoptosis in cancer cells while sparing normal cells . This selective action makes it a promising candidate for cancer therapy .
Properties
IUPAC Name |
(4aS,6aR,6aS,6bR,8aR,9R,10S,12aR,14bS)-9-(hydroxymethyl)-10-[(2S,3R,4S,5S)-4-hydroxy-5-[(2S,3R,4S,5S,6R)-3,4,5-trihydroxy-6-(hydroxymethyl)oxan-2-yl]oxy-3-[(2S,3R,4R,5R,6S)-3,4,5-trihydroxy-6-methyloxan-2-yl]oxyoxan-2-yl]oxy-2,2,6a,6b,9,12a-hexamethyl-1,3,4,5,6,6a,7,8,8a,10,11,12,13,14b-tetradecahydropicene-4a-carboxylic acid | |
---|---|---|
Source | PubChem | |
URL | https://pubchem.ncbi.nlm.nih.gov | |
Description | Data deposited in or computed by PubChem | |
InChI |
InChI=1S/C47H76O17/c1-22-30(50)33(53)35(55)38(60-22)64-37-32(52)26(62-39-36(56)34(54)31(51)25(19-48)61-39)20-59-40(37)63-29-11-12-43(4)27(44(29,5)21-49)10-13-46(7)28(43)9-8-23-24-18-42(2,3)14-16-47(24,41(57)58)17-15-45(23,46)6/h8,22,24-40,48-56H,9-21H2,1-7H3,(H,57,58)/t22-,24-,25+,26-,27+,28+,29-,30-,31+,32-,33+,34-,35+,36+,37+,38-,39-,40-,43-,44-,45+,46+,47-/m0/s1 | |
Source | PubChem | |
URL | https://pubchem.ncbi.nlm.nih.gov | |
Description | Data deposited in or computed by PubChem | |
InChI Key |
SOLICHUQXFAOEP-YDIXZRNLSA-N | |
Source | PubChem | |
URL | https://pubchem.ncbi.nlm.nih.gov | |
Description | Data deposited in or computed by PubChem | |
Canonical SMILES |
CC1C(C(C(C(O1)OC2C(C(COC2OC3CCC4(C(C3(C)CO)CCC5(C4CC=C6C5(CCC7(C6CC(CC7)(C)C)C(=O)O)C)C)C)OC8C(C(C(C(O8)CO)O)O)O)O)O)O)O | |
Source | PubChem | |
URL | https://pubchem.ncbi.nlm.nih.gov | |
Description | Data deposited in or computed by PubChem | |
Isomeric SMILES |
C[C@H]1[C@@H]([C@H]([C@H]([C@@H](O1)O[C@@H]2[C@H]([C@H](CO[C@H]2O[C@H]3CC[C@]4([C@H]([C@]3(C)CO)CC[C@@]5([C@@H]4CC=C6[C@]5(CC[C@@]7([C@H]6CC(CC7)(C)C)C(=O)O)C)C)C)O[C@H]8[C@@H]([C@H]([C@@H]([C@H](O8)CO)O)O)O)O)O)O)O | |
Source | PubChem | |
URL | https://pubchem.ncbi.nlm.nih.gov | |
Description | Data deposited in or computed by PubChem | |
Molecular Formula |
C47H76O17 | |
Source | PubChem | |
URL | https://pubchem.ncbi.nlm.nih.gov | |
Description | Data deposited in or computed by PubChem | |
DSSTOX Substance ID |
DTXSID901315269 | |
Record name | Pulsatilla saponin D | |
Source | EPA DSSTox | |
URL | https://comptox.epa.gov/dashboard/DTXSID901315269 | |
Description | DSSTox provides a high quality public chemistry resource for supporting improved predictive toxicology. | |
Molecular Weight |
913.1 g/mol | |
Source | PubChem | |
URL | https://pubchem.ncbi.nlm.nih.gov | |
Description | Data deposited in or computed by PubChem | |
CAS No. |
68027-15-6 | |
Record name | Pulsatilla saponin D | |
Source | CAS Common Chemistry | |
URL | https://commonchemistry.cas.org/detail?cas_rn=68027-15-6 | |
Description | CAS Common Chemistry is an open community resource for accessing chemical information. Nearly 500,000 chemical substances from CAS REGISTRY cover areas of community interest, including common and frequently regulated chemicals, and those relevant to high school and undergraduate chemistry classes. This chemical information, curated by our expert scientists, is provided in alignment with our mission as a division of the American Chemical Society. | |
Explanation | The data from CAS Common Chemistry is provided under a CC-BY-NC 4.0 license, unless otherwise stated. | |
Record name | Pulsatilla saponin D | |
Source | EPA DSSTox | |
URL | https://comptox.epa.gov/dashboard/DTXSID901315269 | |
Description | DSSTox provides a high quality public chemistry resource for supporting improved predictive toxicology. | |
Q1: What are the primary molecular targets of PSD?
A1: Research suggests that PSD exerts its anticancer effects through multiple mechanisms, primarily by targeting the c-Met receptor tyrosine kinase [, ]. It also disrupts autophagic flux by inhibiting autophagosome-lysosome fusion and lysosomal acidification [, ].
Q2: How does PSD's interaction with c-Met affect cancer cells?
A2: PSD acts as an allosteric inhibitor of c-Met, hindering the activation of downstream signaling pathways crucial for tumor growth, proliferation, and angiogenesis []. This inhibition leads to decreased phosphorylation of key signaling molecules like Akt, mTOR, and p70S6K, ultimately suppressing tumor progression [, ].
Q3: What is the role of PSD in autophagy inhibition, and how does it contribute to its anticancer activity?
A3: PSD disrupts autophagy by blocking autophagosome-lysosome fusion, leading to the accumulation of autophagosomes and p62, a protein normally degraded through autophagy [, ]. This disruption of autophagic flux enhances the anticancer activity of chemotherapeutic agents, suggesting a potential for synergistic therapeutic approaches [, ].
Q4: What is the molecular formula and weight of PSD?
A4: The molecular formula of PSD is C48H76O18, and its molecular weight is 913.1 g/mol.
Q5: Is there any spectroscopic data available for PSD?
A5: Yes, the structural elucidation of PSD has been achieved through various spectroscopic techniques, including FABMS and 1D and 2D NMR []. These techniques provide detailed information about the compound's structure, including the arrangement of its sugar moieties and the stereochemistry of its chiral centers.
Q6: How do structural modifications of PSD impact its activity and toxicity?
A6: Studies on PSD derivatives have revealed valuable insights into its SAR. Modifications to the C-ring, C-28 position, or the sugar moiety at the C-3 position significantly influence its cytotoxicity and hemolytic toxicity []. For instance, some derivatives with modifications at the C-28 position exhibit reduced hemolytic toxicity while maintaining potent anticancer activity [].
Q7: Have any PSD derivatives shown improved pharmacological profiles compared to the parent compound?
A7: Yes, several synthesized PSD derivatives have demonstrated enhanced antiproliferative activity and reduced toxicity compared to the parent compound [, ]. For example, compound 14, a PSD derivative, exhibited significant cytotoxicity against A549 lung cancer cells without exhibiting hemolytic toxicity [].
Q8: Has the metabolism of PSD been studied?
A9: While detailed metabolic profiles are yet to be fully elucidated, studies using LC-MS/MS techniques have identified several metabolites of PSD in rats []. Further research is needed to characterize these metabolites and understand their pharmacological significance.
Q9: What types of cancer cells are most sensitive to PSD?
A10: In vitro studies have demonstrated PSD's antiproliferative activity against a broad range of cancer cell lines, including lung cancer (A549) [, ], hepatocellular carcinoma (SMMC-7721, H22) [, ], breast cancer (MCF-7, MDA-MB-231) [], cervical cancer (HeLa) [], pancreatic cancer [], and glioblastoma (U87-MG, T98G) [, ].
Q10: Has PSD's anticancer efficacy been demonstrated in animal models?
A11: Yes, PSD exhibits significant antitumor activity in various in vivo models. It effectively inhibits tumor growth in xenograft models of hepatocellular carcinoma [, ], lung cancer [], and pancreatic cancer []. These studies provide compelling evidence for PSD's potential as an anticancer therapeutic agent.
Q11: What about clinical trials involving PSD?
A11: While preclinical evidence strongly supports further investigation of PSD, no clinical trials have been conducted to date. Further research is needed to translate these promising findings into clinical settings.
Q12: Are there any strategies being explored to enhance the delivery and targeting of PSD?
A14: Research is ongoing to develop novel drug delivery systems for PSD to improve its bioavailability and target specificity. One such strategy involves using pH-sensitive polymers like Eudragit S100 to create colon-targeted drug delivery systems [].
Q13: How is PSD typically quantified in biological samples?
A15: Liquid chromatography-tandem mass spectrometry (LC-MS/MS) is a sensitive and specific method commonly employed for the quantification of PSD in biological matrices, such as plasma [].
Disclaimer and Information on In-Vitro Research Products
Please be aware that all articles and product information presented on BenchChem are intended solely for informational purposes. The products available for purchase on BenchChem are specifically designed for in-vitro studies, which are conducted outside of living organisms. In-vitro studies, derived from the Latin term "in glass," involve experiments performed in controlled laboratory settings using cells or tissues. It is important to note that these products are not categorized as medicines or drugs, and they have not received approval from the FDA for the prevention, treatment, or cure of any medical condition, ailment, or disease. We must emphasize that any form of bodily introduction of these products into humans or animals is strictly prohibited by law. It is essential to adhere to these guidelines to ensure compliance with legal and ethical standards in research and experimentation.