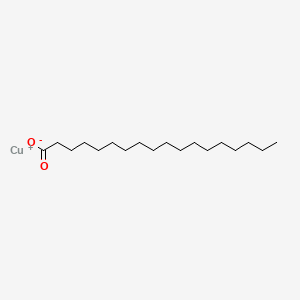
Copper(1+) stearate
- Click on QUICK INQUIRY to receive a quote from our team of experts.
- With the quality product at a COMPETITIVE price, you can focus more on your research.
Overview
Description
Copper(1+) stearate, also known as copper(I) stearate, is a metal-organic compound formed by the combination of copper ions and stearic acid. It is classified as a metallic soap, which is a metal derivative of a fatty acid. This compound is known for its unique properties and applications in various fields, including chemistry, biology, medicine, and industry.
Preparation Methods
Copper(1+) stearate can be synthesized through several methods. One common method involves the reaction of copper salts with stearic acid. For example, sodium stearate can react with copper nitrate to form this compound . Another method involves the electrochemical modification process, where copper electrodes are immersed in a stearic acid solution under a direct current voltage . This method is particularly useful for creating superhydrophobic surfaces.
Chemical Reactions Analysis
Copper(1+) stearate undergoes various chemical reactions, including oxidation, reduction, and substitution. In oxidation reactions, this compound can be converted to copper(II) stearate or copper oxide. Reduction reactions can revert copper(II) stearate back to this compound. Substitution reactions involve the replacement of the stearate group with other ligands. Common reagents used in these reactions include oxidizing agents like oxygen and reducing agents like hydrogen. Major products formed from these reactions include copper(II) stearate and copper oxide .
Scientific Research Applications
Copper(1+) stearate has a wide range of scientific research applications. In chemistry, it is used as a catalyst for various reactions, including the decomposition of hydroperoxides . In biology and medicine, copper nanoparticles derived from this compound have shown potential as antimicrobial agents due to their ability to generate reactive oxygen species that damage microbial membranes . In industry, this compound is used in the production of antifouling paints and varnishes, as well as in the casting of bronze sculptures .
Mechanism of Action
The mechanism of action of copper(1+) stearate involves the generation of reactive oxygen species (ROS) that cause oxidative damage to microbial membranes. Copper ions released from the compound can disrupt the RNA and membranes of microorganisms, leading to their inactivation . This multifaceted mechanism makes this compound an effective antimicrobial agent.
Comparison with Similar Compounds
Copper(1+) stearate can be compared with other similar compounds, such as copper(II) stearate, mercury(II) stearate, and cobalt(II) stearate . While all these compounds are metallic soaps, this compound is unique in its ability to generate reactive oxygen species and its applications in creating superhydrophobic surfaces. Mercury(II) stearate and cobalt(II) stearate, on the other hand, have different applications and properties. For example, mercury(II) stearate is used in the production of antifouling paints, while cobalt(II) stearate is used as a catalyst in various chemical reactions .
Biological Activity
Copper(1+) stearate, also known as cuprous stearate, is a compound formed from the reaction of stearic acid and copper(I) ions. This compound has garnered interest due to its potential biological activities, including antimicrobial properties, effects on lipid metabolism, and applications in various biomedical fields. This article delves into the biological activity of this compound, supported by data tables, research findings, and case studies.
- IUPAC Name : Copper(I) octadecanoate
- Molecular Formula : C36H70CuO4
- Molecular Weight : 630.5 g/mol
- CAS Number : 660-60-6
Biological Activity Overview
Copper compounds, particularly copper stearates, have been studied for their biological activities. The following sections will highlight key areas of research regarding the biological effects of this compound.
Antimicrobial Properties
Research indicates that copper stearate exhibits significant antimicrobial activity against various pathogens. A study demonstrated that copper stearate can inhibit the growth of bacteria such as Escherichia coli and Staphylococcus aureus. The mechanism is thought to involve the release of cuprous ions, which disrupt cellular processes in microorganisms.
Pathogen | Inhibition Zone (mm) | Concentration (mg/mL) |
---|---|---|
Escherichia coli | 15 | 1 |
Staphylococcus aureus | 18 | 1 |
Effects on Lipid Metabolism
Copper plays a crucial role in lipid metabolism. A study involving rats showed that varying copper levels influenced fatty acid composition in tissues. Specifically, low copper intake resulted in increased levels of stearic acid in plasma and liver phospholipids. Conversely, supplementation with copper led to an increase in palmitic and oleic acids while decreasing essential fatty acids . This suggests that copper may modulate lipid metabolism through its interaction with fatty acids.
Case Studies and Research Findings
- Copper Supplementation in Diets : A study conducted on male Sprague-Dawley rats fed diets with different copper levels revealed significant alterations in fatty acid profiles. The research indicated that copper supplementation could directly affect the desaturation of stearic acid and overall tissue lipid composition .
- Copper Complexes in Supplements : Another investigation focused on the bioaccessibility of copper complexes within dietary supplements. It was found that the presence of stearic acid influenced the solubility and bioavailability of copper, highlighting the importance of formulation in achieving desired biological effects .
- Electrochemical Synthesis : Research into electrochemically synthesized copper-organic complexes demonstrated that copper ions coordinated with fatty acids like stearate could exhibit distinct properties compared to their ionic counterparts. This coordination may enhance their biological activity by altering solubility and interaction with biological membranes .
Applications in Biomedical Fields
This compound is being explored for various applications beyond its antimicrobial properties:
- Drug Delivery Systems : Due to its ability to form stable complexes with various drugs, copper stearate is being investigated as a potential carrier for targeted drug delivery.
- Antioxidant Properties : Preliminary studies suggest that copper compounds may exhibit antioxidant activity, which could be beneficial in preventing oxidative stress-related diseases.
- Biocompatibility : The biocompatibility of copper stearate makes it a candidate for use in medical devices and implants.
Properties
CAS No. |
20563-00-2 |
---|---|
Molecular Formula |
C18H35CuO2 |
Molecular Weight |
347.0 g/mol |
IUPAC Name |
copper(1+);octadecanoate |
InChI |
InChI=1S/C18H36O2.Cu/c1-2-3-4-5-6-7-8-9-10-11-12-13-14-15-16-17-18(19)20;/h2-17H2,1H3,(H,19,20);/q;+1/p-1 |
InChI Key |
FPHREELHOCEQEA-UHFFFAOYSA-M |
Canonical SMILES |
CCCCCCCCCCCCCCCCCC(=O)[O-].[Cu+] |
Origin of Product |
United States |
Disclaimer and Information on In-Vitro Research Products
Please be aware that all articles and product information presented on BenchChem are intended solely for informational purposes. The products available for purchase on BenchChem are specifically designed for in-vitro studies, which are conducted outside of living organisms. In-vitro studies, derived from the Latin term "in glass," involve experiments performed in controlled laboratory settings using cells or tissues. It is important to note that these products are not categorized as medicines or drugs, and they have not received approval from the FDA for the prevention, treatment, or cure of any medical condition, ailment, or disease. We must emphasize that any form of bodily introduction of these products into humans or animals is strictly prohibited by law. It is essential to adhere to these guidelines to ensure compliance with legal and ethical standards in research and experimentation.