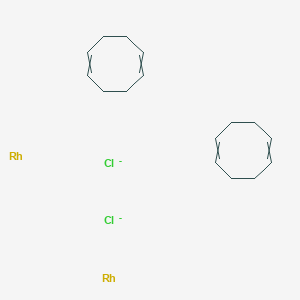
Bis(1,5-cyclooctadiene) dirhodium dichloride
Overview
Description
Bis(1,5-cyclooctadiene)dirhodium(I) dichloride (CAS: 12092-47-6; molecular formula: C₁₆H₂₄Cl₂Rh₂) is a rhodium-based organometallic complex characterized by two 1,5-cyclooctadiene (COD) ligands bridging two rhodium centers via μ-chloro ligands. It appears as an orange-yellow crystalline solid with a melting point of 255°C (decomposition) and is hygroscopic, sparingly soluble in water but soluble in chlorinated solvents like dichloromethane .
This compound is a versatile catalyst, widely employed in asymmetric hydrogenation, hydrofunctionalization reactions (e.g., hydrosilylation), and cross-coupling reactions (e.g., C–C bond formation). Its catalytic activity stems from the labile COD ligands, which dissociate to generate coordinatively unsaturated Rh centers, enabling substrate activation . For example, in hydrosilylation reactions, it produces both α- and β-isomeric products, though with lower selectivity compared to platinum catalysts .
Preparation Methods
The synthesis of Chloro(1,5-cyclooctadiene)rhodium(I) dimer typically involves heating a solution of hydrated rhodium trichloride with 1,5-cyclooctadiene in aqueous ethanol in the presence of sodium carbonate . The reaction can be represented as follows: [ 2 \text{RhCl}_3 \cdot 3\text{H}_2\text{O} + 2 \text{COD} + 2 \text{CH}_3\text{CH}_2\text{OH} + 2 \text{Na}_2\text{CO}_3 \rightarrow [\text{RhCl(COD)}]_2 + 2 \text{CH}_3\text{CHO} + 8 \text{H}_2\text{O} + 2 \text{CO}_2 + 4 \text{NaCl} ]
Chemical Reactions Analysis
Preparation and Structural Basis
The compound is synthesized by reacting hydrated rhodium trichloride with 1,5-cyclooctadiene (COD) in aqueous ethanol, facilitated by sodium carbonate :
Its dimeric structure features two rhodium centers bridged by chloride ligands, each coordinated to a bidentate COD ligand in a square-planar geometry . This configuration enhances its electrophilic reactivity, making it a versatile precursor for catalytic systems.
Asymmetric Hydrogenation
Rh₂(COD)₂Cl₂ is widely used to hydrogenate prochiral alkenes into chiral products with high enantioselectivity . The mechanism involves:
-
Ligand substitution : COD ligands are replaced by chiral phosphines (e.g., BINAP).
-
Substrate coordination : The alkene binds to the Rh center.
-
H₂ activation : Molecular hydrogen dissociates on the metal surface.
-
Stereoselective hydrogen transfer : Yields enantiomerically enriched products.
Substrate | Product | Enantiomeric Excess (ee) | Conditions | Source |
---|---|---|---|---|
α,β-Unsaturated ketones | Chiral alcohols | Up to 99% | 25°C, 1 atm H₂ | |
Prochiral imines | Chiral amines | 85–95% | 50°C, 5 atm H₂ |
Hydrosilylation
The catalyst promotes hydrosilylation of ketones, forming chiral silanes :
Key features :
Hydroboration
Rh₂(COD)₂Cl₂ facilitates cis-hydroboration of terminal alkynes, yielding allylboronates :
Selectivity : >95% cis-addition due to synergic Rh–H and Rh–B interactions .
[2+2+2] Carbocyclization
The catalyst enables enantioselective cyclization of diynes and alkenes into six-membered rings :
Example : Cyclization of 1,6-diynes with norbornene achieves >90% ee under mild conditions .
Reductive Aldol Reaction
Rh₂(COD)₂Cl₂ catalyzes asymmetric reductive aldol reactions, forming β-hydroxy carbonyl compounds :
Conditions : 1 atm H₂, 25°C, with chiral ligands like DuPhos .
Mechanistic Insights
-
Metal–Olefin Interaction : DFT studies reveal stronger Rh–COD bonding in the D₂h symmetry (solid state) compared to D₂ (vapor phase), influencing catalytic activity .
-
Chloride Bridging : The Rh₂Cl₂ core stabilizes transition states during hydrogenation and hydrosilylation .
Property | Rh₂(COD)₂Cl₂ | Ir Analogue | Source |
---|---|---|---|
Metal–olefin bond strength | Moderate | Stronger | |
Catalytic efficiency | High | Slightly lower |
Scientific Research Applications
Chloro(1,5-cyclooctadiene)rhodium(I) dimer is extensively used in scientific research due to its versatility as a catalyst. Some of its applications include:
Catalysis: It is a widely used precursor to homogeneous catalysts, particularly in asymmetric hydrogenation.
Synthesis of Metal Ligands: It is used in the synthesis of other metal ligands for catalysis.
Hydroformylation Reactions: It is modified and coated on the surface of ferrite magnetic nanoparticles for catalyzing hydroformylation reactions of olefins.
Mechanism of Action
The mechanism of action of Chloro(1,5-cyclooctadiene)rhodium(I) dimer involves the formation of adducts with Lewis bases. The molecule consists of a pair of square planar rhodium centers bound to a 1,5-cyclooctadiene and two chloride ligands that are shared between the rhodium centers . This structure allows it to act as an effective catalyst in various chemical reactions.
Comparison with Similar Compounds
Structural and Catalytic Comparisons
Bis(1,5-cyclooctadiene)diiridium(I) Dichloride (CAS: 12112-67-3)
- Structure : Analogous dirhodium complex but with iridium as the metal center.
- Catalytic Activity : Exhibits higher stability under oxidative conditions due to iridium’s resistance to oxidation. Used in enantioselective allylic vinylation with potassium alkenyltrifluoroborates, achieving high enantiomeric ratios (e.g., 86:14 er in spirocyclic indole synthesis) .
- Selectivity : Superior to dirhodium in certain asymmetric transformations, attributed to iridium’s stronger metal-ligand interactions .
Bis(1,5-cyclooctadiene)nickel (CAS: 1295-35-8)
- Structure : Neutral nickel(0) complex with two COD ligands.
- Catalytic Activity: Primarily used in alkene oligomerization and polymerization.
- Limitations : Less effective in hydrogenation or hydrosilylation compared to Rh/Ir complexes.
Dichloro(1,5-cyclooctadiene)palladium(II) (CAS: 12107-56-1)
- Structure: Monomeric Pd(II) complex with one COD ligand.
- Catalytic Activity : Specialized in Heck couplings and C–H activation . The Pd center facilitates oxidative addition steps more readily than Rh or Ir .
- Thermal Stability : Decomposes at lower temperatures (~120°C), limiting high-temperature applications .
Dichloro(1,5-cyclooctadiene)platinum(II)
- Structure: Monomeric Pt(II) complex with one COD ligand.
- Catalytic Activity : Highly selective in hydrosilylation , favoring β-adducts (>99% selectivity with dimethylphenylsilane) due to Pt’s electrophilic character .
- Cost and Availability : Platinum’s higher cost restricts industrial use compared to Rh or Ir complexes .
Performance in Hydrosilylation Reactions
Key Findings :
- Dirhodium dichloride exhibits moderate β-selectivity but generates dehydrogenative coupling byproducts (e.g., entry 14 in Table 2, ).
- Platinum complexes achieve near-exclusive β-selectivity due to stronger electrophilicity, making them preferable for regioselective hydrosilylation .
Stability and Reactivity
Biological Activity
Bis(1,5-cyclooctadiene) dirhodium dichloride , with the chemical formula , is a transition metal complex that has garnered attention in various fields, including catalysis and medicinal chemistry. This article focuses on its biological activity, highlighting its potential therapeutic applications, mechanisms of action, and relevant case studies.
- Molecular Weight : 493.079 g/mol
- CAS Number : 12092-47-6
- IUPAC Name : Bis(1,5-cyclooctadiene) dirhodium(I) dichloride
- Structure : The compound features a dimeric structure where two rhodium centers are coordinated by two 1,5-cyclooctadiene ligands and two chloride ions.
Anticancer Potential
Recent studies have investigated the anticancer properties of this compound. The compound has been shown to exhibit cytotoxic effects in various cancer cell lines. For instance, one study reported that it induced apoptosis in FaDu hypopharyngeal tumor cells, demonstrating a mechanism that appears to be linked to oxidative stress and mitochondrial dysfunction .
Case Study: FaDu Cell Line
Parameter | Value |
---|---|
Cell Line | FaDu |
IC50 | 15 µM |
Mechanism of Action | Induction of apoptosis through ROS generation |
Reference |
The biological activity of this compound is primarily attributed to its ability to generate reactive oxygen species (ROS). These ROS can lead to cellular damage and apoptosis in cancer cells. The compound also interacts with various cellular pathways, including those involved in cell cycle regulation and apoptosis .
Enzyme Inhibition
In addition to its anticancer properties, this compound has been studied for its potential as an enzyme inhibitor. It has shown promise in inhibiting certain metalloproteinases, which are implicated in cancer metastasis and tissue remodeling .
Comparative Analysis with Other Rhodium Complexes
To better understand the biological activity of this compound, it is useful to compare it with other rhodium complexes.
Q & A
Basic Research Questions
Q. What are the primary catalytic applications of bis(1,5-cyclooctadiene) dirhodium dichloride in organic synthesis?
this compound is widely used as a homogeneous catalyst in hydrogenation, hydroformylation, and C–H activation reactions due to its ability to stabilize reactive intermediates via π-coordination of the cyclooctadiene ligand. Researchers should design experiments by selecting substrates compatible with Rh(I) centers and ensuring inert atmospheric conditions to prevent oxidation . Reaction monitoring via GC-MS or NMR is recommended to track catalytic efficiency.
Q. What safety precautions are critical when handling this compound?
The compound is classified as a skin and eye irritant (Category 2/2A). Mandatory precautions include working in a fume hood, wearing nitrile gloves, safety goggles, and lab coats. In case of exposure, flush eyes with water for 15 minutes and wash skin with soap . Storage should be in airtight containers under inert gas (e.g., argon) at 2–8°C to prevent decomposition .
Q. How can researchers verify the purity and structural integrity of this catalyst?
Purity analysis is typically conducted via elemental analysis (C/H/N) and ICP-MS for rhodium content. Structural confirmation requires single-crystal X-ray diffraction or / NMR to validate the coordination of cyclooctadiene ligands and chloride counterions . Contamination with Rh(III) species (e.g., due to oxidation) can be detected via UV-Vis spectroscopy at 450–500 nm .
Advanced Research Questions
Q. How do reaction conditions (solvent, temperature) influence the catalytic activity of this compound?
Solvent polarity and coordinating ability significantly impact catalytic performance. For example, non-polar solvents (e.g., toluene) enhance substrate binding to Rh(I), while polar aprotic solvents (e.g., DMF) may stabilize charged intermediates. Temperature optimization (typically 25–80°C) should balance reaction rate and catalyst stability, as prolonged heating above 100°C risks ligand dissociation and Rh aggregation . Experimental design should include a factorial analysis varying solvent, temperature, and substrate ratios.
Q. What methodologies resolve conflicting reports on enantioselectivity in asymmetric catalysis using this compound?
Discrepancies in enantioselectivity often arise from ligand impurities or trace moisture. Researchers should:
- Purify ligands via column chromatography.
- Use anhydrous solvents (tested with Karl Fischer titration).
- Employ chiral HPLC to confirm enantiomeric excess (ee).
- Conduct control experiments with rigorously dried catalysts to isolate variables .
Q. How can computational chemistry complement experimental studies of this catalyst’s mechanism?
Density Functional Theory (DFT) simulations can model transition states and electron transfer pathways, particularly in C–H activation steps. Researchers should compare computed activation energies with experimental kinetic data (e.g., Arrhenius plots) to validate mechanistic hypotheses. Software like Gaussian or ORCA is recommended, with basis sets (e.g., LANL2DZ) accounting for relativistic effects in Rh .
Q. What strategies mitigate catalyst deactivation during long-term reactions?
Deactivation often results from Rh nanoparticle formation or ligand oxidation. Strategies include:
- Adding stabilizing ligands (e.g., phosphines) to suppress aggregation.
- Using reducing agents (e.g., H) to maintain Rh(I) oxidation state.
- Implementing flow chemistry systems to minimize exposure to oxidative byproducts .
Q. Methodological and Analytical Considerations
Q. How should researchers address the lack of ecological toxicity data for this compound?
While no ecotoxicological data is available, disposal must comply with EPA TSCA regulations. Treat waste with chelating agents (e.g., EDTA) to sequester Rh ions before incineration. Researchers should document disposal protocols to prevent environmental release .
Q. What spectroscopic techniques are optimal for in situ monitoring of catalytic intermediates?
Operando IR spectroscopy tracks ligand vibrations (e.g., C=C stretches of cyclooctadiene at 1600–1650 cm). XAS (X-ray Absorption Spectroscopy) at Rh K-edge (23.2 keV) provides oxidation state and coordination geometry data. Pair these with stopped-flow techniques for time-resolved analysis .
Q. Theoretical and Framework Integration
Q. How can researchers align studies of this catalyst with broader organometallic theory?
Link experiments to concepts like the 18-electron rule, oxidative addition/reductive elimination, and ligand steric effects (e.g., Tolman’s cone angle). For example, cyclooctadiene’s labile π-bonding allows dynamic ligand exchange, a property critical in catalytic cycles. Theoretical frameworks should guide hypothesis generation and data interpretation .
Properties
IUPAC Name |
cycloocta-1,5-diene;rhodium;dichloride | |
---|---|---|
Details | Computed by LexiChem 2.6.6 (PubChem release 2019.06.18) | |
Source | PubChem | |
URL | https://pubchem.ncbi.nlm.nih.gov | |
Description | Data deposited in or computed by PubChem | |
InChI |
InChI=1S/2C8H12.2ClH.2Rh/c2*1-2-4-6-8-7-5-3-1;;;;/h2*1-2,7-8H,3-6H2;2*1H;;/p-2 | |
Details | Computed by InChI 1.0.5 (PubChem release 2019.06.18) | |
Source | PubChem | |
URL | https://pubchem.ncbi.nlm.nih.gov | |
Description | Data deposited in or computed by PubChem | |
InChI Key |
PDJQCHVMABBNQW-UHFFFAOYSA-L | |
Details | Computed by InChI 1.0.5 (PubChem release 2019.06.18) | |
Source | PubChem | |
URL | https://pubchem.ncbi.nlm.nih.gov | |
Description | Data deposited in or computed by PubChem | |
Canonical SMILES |
C1CC=CCCC=C1.C1CC=CCCC=C1.[Cl-].[Cl-].[Rh].[Rh] | |
Details | Computed by OEChem 2.1.5 (PubChem release 2019.06.18) | |
Source | PubChem | |
URL | https://pubchem.ncbi.nlm.nih.gov | |
Description | Data deposited in or computed by PubChem | |
Molecular Formula |
C16H24Cl2Rh2-2 | |
Details | Computed by PubChem 2.1 (PubChem release 2019.06.18) | |
Source | PubChem | |
URL | https://pubchem.ncbi.nlm.nih.gov | |
Description | Data deposited in or computed by PubChem | |
Molecular Weight |
493.1 g/mol | |
Details | Computed by PubChem 2.1 (PubChem release 2021.05.07) | |
Source | PubChem | |
URL | https://pubchem.ncbi.nlm.nih.gov | |
Description | Data deposited in or computed by PubChem | |
Disclaimer and Information on In-Vitro Research Products
Please be aware that all articles and product information presented on BenchChem are intended solely for informational purposes. The products available for purchase on BenchChem are specifically designed for in-vitro studies, which are conducted outside of living organisms. In-vitro studies, derived from the Latin term "in glass," involve experiments performed in controlled laboratory settings using cells or tissues. It is important to note that these products are not categorized as medicines or drugs, and they have not received approval from the FDA for the prevention, treatment, or cure of any medical condition, ailment, or disease. We must emphasize that any form of bodily introduction of these products into humans or animals is strictly prohibited by law. It is essential to adhere to these guidelines to ensure compliance with legal and ethical standards in research and experimentation.