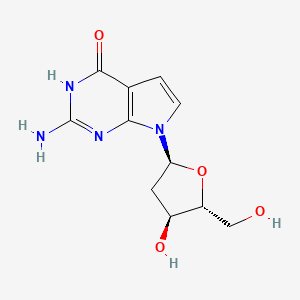
7-Deaza-2'-dG
- Click on QUICK INQUIRY to receive a quote from our team of experts.
- With the quality product at a COMPETITIVE price, you can focus more on your research.
Overview
Description
7-Deaza-2’-deoxyguanosine is a modified nucleoside in which the nitrogen atom at the seventh position of the guanine base is replaced by a carbon-hydrogen group. This modification results in the inability of the nucleoside to form a hydrogen bond at position seven, which can significantly affect the structure and function of DNA and RNA .
Preparation Methods
Synthetic Routes and Reaction Conditions
The synthesis of 7-Deaza-2’-deoxyguanosine typically involves the halogenation of nucleobases followed by nucleophilic substitution reactions. One common method includes the use of iodine oxidation or 0.5M CSO in anhydrous acetonitrile with a three-minute oxidation time . Another approach involves the use of dithiothreitol, isopropyl-β-D-thiogalactopyranoside, kanamycin sulfate, and ampicillin in a reaction buffer at pH 7.1, with specific concentrations of potassium chloride, magnesium chloride, and dithiothreitol .
Industrial Production Methods
Industrial production methods for 7-Deaza-2’-deoxyguanosine are not extensively documented, but they likely involve large-scale synthesis using similar chemical reactions and conditions as those used in laboratory settings. The use of automated synthesizers and high-throughput purification techniques would be essential for efficient production.
Chemical Reactions Analysis
Types of Reactions
7-Deaza-2’-deoxyguanosine undergoes various chemical reactions, including:
Oxidation: The compound can be oxidized using iodine or other oxidizing agents.
Reduction: Reduction reactions can be performed using reducing agents like dithiothreitol.
Substitution: Nucleophilic substitution reactions are common, where halogenated intermediates are replaced by nucleophiles.
Common Reagents and Conditions
Oxidizing Agents: Iodine, 0.5M CSO in anhydrous acetonitrile.
Reducing Agents: Dithiothreitol.
Nucleophiles: Various nucleophiles can be used depending on the desired substitution.
Major Products
The major products formed from these reactions include various derivatives of 7-Deaza-2’-deoxyguanosine, such as 7-deaza-2’-deoxyadenosine and other modified nucleosides .
Scientific Research Applications
7-Deaza-2'-deoxyguanosine (7-Deaza-dG) is a modified nucleobase analog of guanine, where the nitrogen at the 7th position of the purine ring is replaced with a carbon atom . This modification alters the electronic and structural properties of the nucleobase, leading to a variety of applications in scientific research .
Scientific Research Applications
Structural Studies of DNA and DNA-Protein Interactions
- Enzyme Interactions: 7-Deaza-dG is utilized in physical studies concerning the interaction between enzymes and DNA . The absence of the nitrogen atom at the 7th position affects hydrogen bonding, which can be crucial for enzyme recognition and binding .
- Triplex DNA: Researchers use 7-Deaza-dG to study the formation and structure of triplex DNA . Introducing 7-deaza purine monomers into oligonucleotides can stabilize them relative to oligonucleotides containing adenine and guanine .
- Minor Groove Interactions: Standard nucleobases possess unshared electron pairs projecting into the minor groove of duplex DNA . Enzymes like polymerases and reverse transcriptases may use hydrogen bond donating groups to contact hydrogen bond acceptors in the minor groove, and 7-Deaza-dG modifications can influence these interactions .
PCR and Oligonucleotide Synthesis
- GC-Rich Templates: Partial substitution of dGTP with 7-Deaza-dGTP in PCR can enhance the yield of reaction products, especially for GC-rich templates that contain strong secondary structures . The modification helps in reducing secondary structures that can inhibit PCR amplification .
- Oligonucleotide Stability: Unlike guanine, 7-Deaza-dG does not lead to aggregation, which means that G-rich oligos can be easily synthesized and isolated .
- Fluorescence Quenching: 5'-fluorescein-modified oligonucleotides containing 7-Deaza-dG are less quenched compared to those with natural guanine bases, making them useful in fluorescence-based detection assays .
Antiviral Research
- 7DMA Synthesis: 7-Deaza-dG is a precursor in synthesizing antiviral agents like 7DMA (7-Deaza-2-methyladenine) . 7DMA has shown moderate activity against the emerging flavivirus USUV (Usutu virus) in vitro .
- Antiviral Activity Assessment: 7DMA has been tested against flaviviruses like ZIKV (Zika virus) and WNV (West Nile virus), which have proven more susceptible to 7DMA . Studies have indicated that 7DMA is less toxic and more effective in reducing viral replication in neuronal cells, which are primary target cells for USUV .
Inhibition of DNA Repair Enzymes
- hOGG1 Inhibition: 8-Halogenated-7-deaza-dG derivatives have been designed and synthesized as 8-oxo-dG analogs and evaluated for their chemical and biological properties . These derivatives resemble 8-oxo-dG in shape and preference for syn-conformation but do not form Hoogsteen base pairs with adenine .
- Glycosidase Substrates: 8-halogenated-7-deaza-dG derivatives in duplex DNA, particularly 8-Cl-7-deaza-dG, serve as glycosidase substrates for Fpg and strong binders to hOGG1 . They act as competitive inhibitors for the glycosidase activity of hOGG1, which excises 8-oxo-dG in duplex DNA .
- hMTH1 Targeting: 7-deaza-dGTP and 8-halogenated-7-deaza-dGTP derivatives have been synthesized and tested for reactivity with hMTH1, showing competitive inhibitory activity against 8-oxo-dGTP hydrolysis . These compounds may exhibit antitumor activity by targeting hMTH1 .
Mechanism of Action
7-Deaza-2’-deoxyguanosine exerts its effects by altering the hydrogen bonding patterns in nucleic acids. This modification can affect the stability and conformation of DNA and RNA, leading to changes in their biological functions. The compound targets specific nucleic acid sequences and can interfere with processes such as replication, transcription, and translation .
Comparison with Similar Compounds
Similar Compounds
7-Deaza-2’-deoxyadenosine: Similar to 7-Deaza-2’-deoxyguanosine, but with modifications at the adenine base.
2’-Deoxy-7-cyano-7-deazaguanosine: Another modified nucleoside with a cyano group at the seventh position.
2’-Deoxy-7-amido-7-deazaguanosine: Contains an amido group at the seventh position.
Uniqueness
7-Deaza-2’-deoxyguanosine is unique due to its specific modification at the seventh position, which significantly alters its hydrogen bonding capabilities and affects the overall structure and function of nucleic acids. This makes it a valuable tool in various scientific and medical research applications .
Biological Activity
7-Deaza-2'-deoxyguanosine (7-deaza-dG) is a modified nucleoside that has garnered attention in the field of molecular biology and medicinal chemistry due to its unique structural properties and biological activities. This compound, a derivative of deoxyguanosine, replaces the nitrogen atom at the 7-position of guanine with a carbon atom, which alters its electronic properties and interactions with DNA and proteins. This article explores the biological activity of 7-deaza-dG, focusing on its synthesis, incorporation into DNA, and potential therapeutic applications.
Synthesis and Derivatives
7-Deaza-dG can be synthesized through various chemical pathways, often involving the modification of existing nucleoside structures. Recent studies have explored derivatives such as halogenated forms (e.g., 8-halogenated-7-deaza-dG), which exhibit distinct biological properties. These derivatives are synthesized by reacting acetylated 7-deaza-dG with N-halogenated succinimides, leading to compounds that can be incorporated into oligonucleotides for further study .
Base Pairing Properties
The incorporation of 7-deaza-dG into DNA has been shown to affect base pairing dynamics. It typically forms stable Watson-Crick base pairs with cytosine (C), although the absence of the 7-N atom alters the hydrogen bonding capabilities and cation-binding sites in the major groove of DNA. This modification can influence the overall stability and flexibility of DNA structures .
Table 1: Base Pairing Characteristics of 7-Deaza-dG
Property | Value/Observation |
---|---|
Base Pairing Type | Watson-Crick with C |
Stability Comparison | Less stable than unmodified G |
Cation Binding | Reduced due to loss of N-H bond at position 7 |
Enzyme Interactions
Research has demonstrated that 7-deaza-dG and its derivatives can act as competitive inhibitors for various DNA repair enzymes, such as hOGG1 (human 8-oxoguanine DNA glycosylase). For instance, halogenated derivatives showed significant inhibition of glycosidase activity, suggesting their potential as therapeutic agents targeting DNA repair mechanisms .
Case Study: Inhibition Mechanism
In a recent study, S-halogenated-7-deaza-dG derivatives were evaluated for their ability to inhibit hMTH1 (human mutT homolog 1) activity. These compounds displayed competitive inhibition against S-oxo-dGTP hydrolysis by hMTH1, indicating that they could effectively interfere with nucleotide repair processes in cancer cells. The halogenated versions were found to be poorly hydrolyzed by hMTH1 while still exhibiting significant inhibitory activity, highlighting their potential as antitumor agents .
Thermodynamic Stability
The thermodynamic properties of DNA containing 7-deaza-dG have been characterized using techniques such as UV thermal melting and differential scanning calorimetry (DSC). These studies indicate that the presence of 7-deaza-dG can destabilize duplex DNA compared to unmodified counterparts. The dynamic structure changes due to altered hydration and cation organization around the modified base contribute to this destabilization .
Table 2: Thermodynamic Properties of Modified DNA
Modification | Melting Temperature (Tm) | Stability Relative to Unmodified |
---|---|---|
Unmodified dG | X °C | Baseline |
7-Deaza-dG | Y °C | Decreased |
S-Cl-7-deaza-dG | Z °C | Similar to unmodified |
Therapeutic Applications
The unique properties of 7-deaza-dG derivatives suggest potential applications in cancer therapy. Their ability to inhibit key enzymes involved in DNA repair could make them useful in sensitizing cancer cells to chemotherapy or radiotherapy. Additionally, their reduced incorporation into DNA during replication minimizes potential side effects associated with traditional nucleoside analogs.
Properties
Molecular Formula |
C11H14N4O4 |
---|---|
Molecular Weight |
266.25 g/mol |
IUPAC Name |
2-amino-7-[(2S,4S,5R)-4-hydroxy-5-(hydroxymethyl)oxolan-2-yl]-3H-pyrrolo[2,3-d]pyrimidin-4-one |
InChI |
InChI=1S/C11H14N4O4/c12-11-13-9-5(10(18)14-11)1-2-15(9)8-3-6(17)7(4-16)19-8/h1-2,6-8,16-17H,3-4H2,(H3,12,13,14,18)/t6-,7+,8-/m0/s1 |
InChI Key |
PFCLMNDDPTZJHQ-RNJXMRFFSA-N |
Isomeric SMILES |
C1[C@@H]([C@H](O[C@@H]1N2C=CC3=C2N=C(NC3=O)N)CO)O |
Canonical SMILES |
C1C(C(OC1N2C=CC3=C2N=C(NC3=O)N)CO)O |
Origin of Product |
United States |
Disclaimer and Information on In-Vitro Research Products
Please be aware that all articles and product information presented on BenchChem are intended solely for informational purposes. The products available for purchase on BenchChem are specifically designed for in-vitro studies, which are conducted outside of living organisms. In-vitro studies, derived from the Latin term "in glass," involve experiments performed in controlled laboratory settings using cells or tissues. It is important to note that these products are not categorized as medicines or drugs, and they have not received approval from the FDA for the prevention, treatment, or cure of any medical condition, ailment, or disease. We must emphasize that any form of bodily introduction of these products into humans or animals is strictly prohibited by law. It is essential to adhere to these guidelines to ensure compliance with legal and ethical standards in research and experimentation.