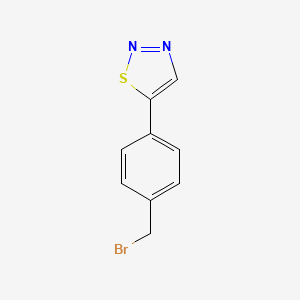
5-(4-(Bromomethyl)phenyl)-1,2,3-thiadiazole
- Click on QUICK INQUIRY to receive a quote from our team of experts.
- With the quality product at a COMPETITIVE price, you can focus more on your research.
Overview
Description
5-(4-(Bromomethyl)phenyl)-1,2,3-thiadiazole is an organic compound that belongs to the class of thiadiazoles Thiadiazoles are heterocyclic compounds containing both sulfur and nitrogen atoms in a five-membered ring This particular compound is characterized by the presence of a bromomethyl group attached to a phenyl ring, which is further connected to the thiadiazole ring
Preparation Methods
Synthetic Routes and Reaction Conditions
The synthesis of 5-(4-(Bromomethyl)phenyl)-1,2,3-thiadiazole typically involves the cyclization of appropriate precursors under specific conditions. One common method includes the reaction of 4-(bromomethyl)benzenediazonium chloride with thiourea, followed by cyclization to form the thiadiazole ring. The reaction is usually carried out in an acidic medium, such as hydrochloric acid, and at elevated temperatures to facilitate the cyclization process.
Industrial Production Methods
While specific industrial production methods for this compound are not widely documented, the general approach would involve scaling up the laboratory synthesis methods. This would include optimizing reaction conditions, such as temperature, pressure, and solvent choice, to ensure high yield and purity of the final product. Continuous flow reactors and other advanced manufacturing techniques may be employed to enhance efficiency and scalability.
Chemical Reactions Analysis
Types of Reactions
5-(4-(Bromomethyl)phenyl)-1,2,3-thiadiazole undergoes various chemical reactions, including:
Substitution Reactions: The bromomethyl group can participate in nucleophilic substitution reactions, where the bromine atom is replaced by other nucleophiles.
Oxidation and Reduction: The compound can undergo oxidation and reduction reactions, although specific conditions and reagents would depend on the desired transformation.
Cyclization Reactions: The thiadiazole ring can participate in further cyclization reactions to form more complex heterocyclic structures.
Common Reagents and Conditions
Nucleophilic Substitution: Common reagents include sodium azide, potassium cyanide, and various amines. Reactions are typically carried out in polar aprotic solvents like dimethylformamide (DMF) or dimethyl sulfoxide (DMSO).
Oxidation: Reagents such as potassium permanganate or chromium trioxide can be used under acidic or basic conditions.
Reduction: Reducing agents like lithium aluminum hydride (LiAlH4) or sodium borohydride (NaBH4) are commonly employed.
Major Products
The major products formed from these reactions depend on the specific reagents and conditions used. For example, nucleophilic substitution with sodium azide would yield the corresponding azide derivative, while oxidation with potassium permanganate could lead to the formation of carboxylic acids or other oxidized products.
Scientific Research Applications
5-(4-(Bromomethyl)phenyl)-1,2,3-thiadiazole has several scientific research applications:
Chemistry: It is used as a building block in the synthesis of more complex heterocyclic compounds and as a precursor in various organic reactions.
Medicine: Research is ongoing to explore its potential as a therapeutic agent, particularly in the treatment of infectious diseases and cancer.
Industry: It is used in the development of advanced materials, such as polymers and coatings, due to its unique chemical properties.
Mechanism of Action
The mechanism of action of 5-(4-(Bromomethyl)phenyl)-1,2,3-thiadiazole depends on its specific application. In biological systems, it may interact with various molecular targets, such as enzymes or receptors, to exert its effects. The bromomethyl group can act as an electrophile, forming covalent bonds with nucleophilic sites on proteins or other biomolecules. This interaction can lead to the inhibition of enzyme activity or modulation of signaling pathways, contributing to its biological activity.
Comparison with Similar Compounds
Similar Compounds
5-Phenyl-1,2,3-thiadiazole: Lacks the bromomethyl group, resulting in different reactivity and applications.
4-(Bromomethyl)benzoic acid: Contains a bromomethyl group but lacks the thiadiazole ring, leading to different chemical properties and uses.
1,3,4-Thiadiazole derivatives: Various derivatives with different substituents on the thiadiazole ring, each with unique properties and applications.
Uniqueness
5-(4-(Bromomethyl)phenyl)-1,2,3-thiadiazole is unique due to the presence of both the bromomethyl group and the thiadiazole ring. This combination imparts distinct chemical reactivity and potential for diverse applications in research and industry. Its ability to undergo various chemical transformations and interact with biological targets makes it a valuable compound for scientific exploration.
Biological Activity
5-(4-(Bromomethyl)phenyl)-1,2,3-thiadiazole is a member of the thiadiazole family, which has garnered attention due to its diverse biological activities. This article provides a comprehensive overview of its biological properties, focusing on its anticancer, antimicrobial, and anticonvulsant activities.
Structural Characteristics
The compound features a thiadiazole ring, which is a five-membered heterocyclic structure containing two nitrogen atoms and one sulfur atom. The bromomethyl group attached to the phenyl ring enhances its reactivity and biological potential.
Anticancer Activity
Recent studies have demonstrated that 1,2,3-thiadiazole derivatives exhibit significant anticancer properties. The mechanism often involves the induction of apoptosis in cancer cells.
Case Studies and Findings
- Cytotoxicity Assays : Research indicates that derivatives of this compound show varying degrees of cytotoxicity against different cancer cell lines. For instance, in vitro studies revealed that certain derivatives had IC50 values ranging from 2.32 µg/mL to 10.10 µg/mL against breast adenocarcinoma (MCF-7) and hepatocellular carcinoma (HepG2) cell lines .
- Mechanism of Action : The anticancer activity is attributed to the ability of these compounds to disrupt cellular processes leading to apoptosis. For example, compounds with specific substitutions on the thiadiazole ring demonstrated enhanced cytotoxic effects by targeting key proteins involved in cell survival pathways .
Compound | Cell Line | IC50 (µg/mL) | Mechanism |
---|---|---|---|
4i | MCF-7 | 2.32 | Apoptosis induction |
4f | HepG2 | 5.36 | Cell cycle arrest |
4e | MCF-7 | 10.10 | Inhibition of proliferation |
Antimicrobial Activity
Thiadiazole derivatives have also been explored for their antimicrobial properties. The broad-spectrum activity against various pathogens makes them promising candidates for new antimicrobial agents.
Research Insights
- Antibacterial and Antifungal Properties : Thiadiazoles have shown effectiveness against both bacterial and fungal strains. For instance, compounds have been reported to inhibit the growth of Staphylococcus aureus and Candida albicans with minimum inhibitory concentrations (MICs) comparable to standard antibiotics .
- Mechanism : The antimicrobial action is believed to stem from the disruption of microbial cell membranes and interference with metabolic pathways essential for pathogen survival .
Anticonvulsant Activity
The anticonvulsant potential of thiadiazole derivatives has been a focal point in neurological research.
Key Findings
- In Vivo Studies : Compounds were evaluated using models such as maximal electroshock seizure (MES) tests, demonstrating significant anticonvulsant effects comparable to established drugs like phenytoin .
- Structure-Activity Relationship (SAR) : Modifications on the thiadiazole ring influence anticonvulsant efficacy. For example, certain substitutions enhance lipophilicity and improve binding affinity to GABA receptors .
Compound | Test Method | Efficacy |
---|---|---|
Compound A | MES | Significant protection |
Compound B | Phenobarbital test | Moderate protection |
Properties
CAS No. |
154586-22-8 |
---|---|
Molecular Formula |
C9H7BrN2S |
Molecular Weight |
255.14 g/mol |
IUPAC Name |
5-[4-(bromomethyl)phenyl]thiadiazole |
InChI |
InChI=1S/C9H7BrN2S/c10-5-7-1-3-8(4-2-7)9-6-11-12-13-9/h1-4,6H,5H2 |
InChI Key |
TWFBWCABIZOZMV-UHFFFAOYSA-N |
Canonical SMILES |
C1=CC(=CC=C1CBr)C2=CN=NS2 |
Origin of Product |
United States |
Disclaimer and Information on In-Vitro Research Products
Please be aware that all articles and product information presented on BenchChem are intended solely for informational purposes. The products available for purchase on BenchChem are specifically designed for in-vitro studies, which are conducted outside of living organisms. In-vitro studies, derived from the Latin term "in glass," involve experiments performed in controlled laboratory settings using cells or tissues. It is important to note that these products are not categorized as medicines or drugs, and they have not received approval from the FDA for the prevention, treatment, or cure of any medical condition, ailment, or disease. We must emphasize that any form of bodily introduction of these products into humans or animals is strictly prohibited by law. It is essential to adhere to these guidelines to ensure compliance with legal and ethical standards in research and experimentation.