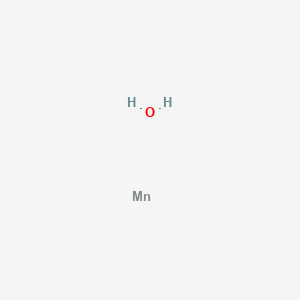
Manganese(1+), hydroxy-
- Click on QUICK INQUIRY to receive a quote from our team of experts.
- With the quality product at a COMPETITIVE price, you can focus more on your research.
Overview
Description
Manganese(1+), hydroxy- is a chemical compound with the formula Mn(OH). It is a manganese ion in the +1 oxidation state bonded to a hydroxide ion. Manganese is a transition metal with the atomic number 25 and is known for its various oxidation states, which contribute to its diverse chemical behavior .
Preparation Methods
Synthetic Routes and Reaction Conditions
Manganese(1+), hydroxy- can be synthesized through several methods. One common approach involves the reaction of manganese(II) salts with a strong base, such as sodium hydroxide, under controlled conditions. The reaction typically proceeds as follows: [ \text{Mn}^{2+} + 2\text{OH}^- \rightarrow \text{Mn(OH)}_2 ] This reaction results in the formation of manganese(II) hydroxide, which can be further processed to obtain manganese(1+), hydroxy- through reduction reactions .
Industrial Production Methods
Industrial production of manganese(1+), hydroxy- often involves high-pressure and high-temperature synthesis techniques. For example, the use of a Walker-type multianvil apparatus allows for the precise control of reaction conditions, leading to the formation of high-purity manganese hydroxide halides .
Chemical Reactions Analysis
Types of Reactions
Manganese(1+), hydroxy- undergoes various chemical reactions, including oxidation, reduction, and substitution reactions. Some common reactions include:
Oxidation: Manganese(1+), hydroxy- can be oxidized to higher oxidation states, such as manganese(II) or manganese(IV), in the presence of oxidizing agents.
Reduction: It can be reduced to manganese metal or lower oxidation states using reducing agents.
Substitution: The hydroxide ion can be substituted with other ligands, such as halides or organic groups.
Common Reagents and Conditions
Common reagents used in these reactions include sodium hydroxide, potassium permanganate, and various organic ligands. Reaction conditions typically involve controlled temperatures and pH levels to ensure the desired product formation .
Major Products Formed
Major products formed from these reactions include manganese oxides, manganese halides, and various manganese-organic complexes. These products have diverse applications in different fields .
Scientific Research Applications
Manganese(1+), hydroxy- has numerous scientific research applications across various fields:
Mechanism of Action
The mechanism of action of manganese(1+), hydroxy- involves its interaction with various molecular targets and pathways. In biological systems, manganese ions can act as cofactors for enzymes, influencing their activity and function. Manganese-induced neurotoxicity, for example, involves oxidative stress, mitochondrial dysfunction, and protein misfolding, leading to neuroinflammation and neurodegeneration .
Comparison with Similar Compounds
Similar Compounds
Similar compounds to manganese(1+), hydroxy- include manganese(II) hydroxide, manganese(IV) oxide, and manganese halides. These compounds share some chemical properties but differ in their oxidation states and reactivity .
Uniqueness
Manganese(1+), hydroxy- is unique due to its specific oxidation state and the resulting chemical behavior. Its ability to undergo various redox reactions and form diverse complexes makes it a valuable compound in both research and industrial applications .
Biological Activity
Manganese(1+), hydroxy- (H₂MnO), is a compound of manganese that exhibits significant biological activity, particularly in relation to its role as an essential trace element in various physiological processes. This article explores its biological effects, mechanisms of action, and relevant case studies, supported by data tables and research findings.
Overview of Manganese in Biological Systems
Manganese is crucial for human health, functioning primarily as a cofactor for numerous enzymes involved in metabolic processes. The oxidation states of manganese, particularly Mn²⁺ and Mn³⁺, play distinct roles in biological systems. Mn²⁺ is the predominant form in cells and is essential for the activity of manganese superoxide dismutase (MnSOD), which protects against oxidative stress by catalyzing the dismutation of superoxide radicals into hydrogen peroxide and oxygen .
1. Enzymatic Functions:
Manganese serves as a cofactor for various enzymes, including:
- MnSOD: Detoxifies superoxide radicals.
- Manganese catalase: Converts hydrogen peroxide into water and oxygen, reducing oxidative stress .
- Other metalloenzymes: Involved in metabolic pathways such as amino acid metabolism and energy production.
2. Reactive Oxygen Species (ROS) Modulation:
Manganese can influence ROS levels within cells. While Mn²⁺ generally exhibits antioxidant properties, Mn³⁺ has been associated with increased oxidative stress and cytotoxicity due to its higher reactivity . This duality underscores the importance of manganese homeostasis in cellular health.
Biological Activity Data
The following table summarizes key findings related to the biological activity of manganese(1+), hydroxy-:
Case Studies
Case Study 1: Manganese and Neurotoxicity
A study investigated the effects of different manganese oxidation states on neuroblastoma cells. It was found that while Mn²⁺ did not significantly alter cell viability, Mn³⁺ exposure led to increased oxidative stress and cell death due to enhanced ROS generation . This study illustrates the importance of oxidation state in determining manganese's biological effects.
Case Study 2: Manganese in Bone Health
Research focusing on the role of manganese in bone health demonstrated that it significantly affects osteogenic differentiation and proliferation of bone marrow mesenchymal stem cells. Concentration-dependent effects were noted, suggesting that both low and high levels of manganese can influence bone density and structure .
Properties
CAS No. |
95118-37-9 |
---|---|
Molecular Formula |
H2MnO |
Molecular Weight |
72.953 g/mol |
IUPAC Name |
manganese;hydrate |
InChI |
InChI=1S/Mn.H2O/h;1H2 |
InChI Key |
BZDIAFGKSAYYFC-UHFFFAOYSA-N |
Canonical SMILES |
O.[Mn] |
Origin of Product |
United States |
Disclaimer and Information on In-Vitro Research Products
Please be aware that all articles and product information presented on BenchChem are intended solely for informational purposes. The products available for purchase on BenchChem are specifically designed for in-vitro studies, which are conducted outside of living organisms. In-vitro studies, derived from the Latin term "in glass," involve experiments performed in controlled laboratory settings using cells or tissues. It is important to note that these products are not categorized as medicines or drugs, and they have not received approval from the FDA for the prevention, treatment, or cure of any medical condition, ailment, or disease. We must emphasize that any form of bodily introduction of these products into humans or animals is strictly prohibited by law. It is essential to adhere to these guidelines to ensure compliance with legal and ethical standards in research and experimentation.