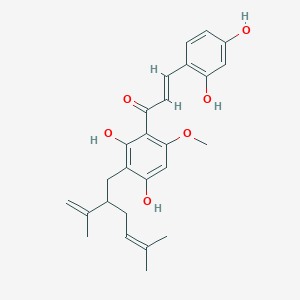
Kuraridin
Overview
Description
KURAIDIN is a chemical compound with the molecular formula C26H30O6 . It contains a total of 62 atoms, including 30 hydrogen atoms, 26 carbon atoms, and 6 oxygen atoms . The structure of KURAIDIN includes 33 non-hydrogen bonds, 16 multiple bonds, 9 rotatable bonds, 4 double bonds, 12 aromatic bonds, 2 six-membered rings, 1 ketone (aromatic), 4 aromatic hydroxyl groups, and 1 aromatic ether
Mechanism of Action
Target of Action
Kuraridine, a prenylated flavonol extracted from the roots of Sophora flavescens , primarily targets the cGMP specific phosphodiesterase type 5 (PDE5) . PDE5 is an enzyme that breaks down cGMP, a molecule that plays a crucial role in vasodilation and smooth muscle relaxation .
Mode of Action
Kuraridine exhibits potent inhibitory activity against cGMP PDE5 . By inhibiting PDE5, Kuraridine prevents the breakdown of cGMP, leading to an increase in cGMP levels . This increase in cGMP enhances vasodilation and smooth muscle relaxation . Additionally, Kuraridine has been found to induce pronounced chloride inward currents in the absence of GABA .
Biochemical Pathways
The primary biochemical pathway affected by Kuraridine is the cGMP-PDE5 pathway . By inhibiting PDE5, Kuraridine increases the levels of cGMP, which in turn activates protein kinase G (PKG). PKG then phosphorylates multiple targets, leading to smooth muscle relaxation and vasodilation .
Pharmacokinetics
It’s known that kuraridine is soluble in dmso , which suggests that it may have good bioavailability
Result of Action
The molecular and cellular effects of Kuraridine’s action primarily involve the relaxation of smooth muscles and vasodilation . These effects are due to the increased levels of cGMP resulting from the inhibition of PDE5 . Additionally, Kuraridine has been found to induce chloride inward currents in the absence of GABA , suggesting potential effects on neuronal signaling.
Biochemical Analysis
Biochemical Properties
Kuraridine plays a crucial role in biochemical reactions, primarily through its inhibitory action on cyclic guanosine monophosphate (cGMP) specific phosphodiesterase type 5 (PDE5). The inhibition of PDE5 by Kuraridine has an IC50 value of 0.64 μM . This inhibition is selective, showing 2.0- and 12.9-fold selectivity over PDE3 and PDE4, respectively . Kuraridine also induces chloride inward currents in the absence of gamma-aminobutyric acid (GABA), enhancing GABA-induced currents in Xenopus oocytes expressing GABA A receptors .
Cellular Effects
Kuraridine influences various cellular processes, including cell signaling pathways, gene expression, and cellular metabolism. It has been shown to induce chloride inward currents, which can affect cellular excitability and signaling . Additionally, Kuraridine’s inhibition of PDE5 can lead to increased levels of cGMP, impacting various downstream signaling pathways and cellular functions .
Molecular Mechanism
At the molecular level, Kuraridine exerts its effects primarily through the inhibition of PDE5. This inhibition prevents the breakdown of cGMP, leading to elevated cGMP levels within cells . The increased cGMP levels can activate protein kinase G (PKG), which in turn can phosphorylate various target proteins, leading to changes in cellular functions and gene expression . Kuraridine’s interaction with GABA A receptors also suggests a role in modulating neurotransmitter signaling .
Temporal Effects in Laboratory Settings
In laboratory settings, the effects of Kuraridine can change over time. Kuraridine is stable when stored as a powder at -20°C for up to three years and in solution at -80°C for up to six months . Over time, Kuraridine’s inhibitory effects on PDE5 and its impact on cellular functions can be observed in both in vitro and in vivo studies . Long-term exposure to Kuraridine may lead to sustained changes in cellular signaling and function .
Dosage Effects in Animal Models
The effects of Kuraridine vary with different dosages in animal models. At lower doses, Kuraridine effectively inhibits PDE5 without causing significant adverse effects . At higher doses, Kuraridine may exhibit toxic effects, including potential impacts on liver and kidney function . It is essential to determine the optimal dosage to balance efficacy and safety in animal studies .
Metabolic Pathways
Kuraridine is involved in metabolic pathways related to its inhibitory action on PDE5. The inhibition of PDE5 by Kuraridine affects the cGMP signaling pathway, leading to changes in metabolic flux and metabolite levels . Kuraridine’s interaction with other enzymes and cofactors in these pathways can further modulate its metabolic effects .
Transport and Distribution
Within cells and tissues, Kuraridine is transported and distributed through various mechanisms. It interacts with specific transporters and binding proteins that facilitate its uptake and localization . Kuraridine’s distribution can affect its concentration in different cellular compartments, influencing its bioactivity and efficacy .
Subcellular Localization
Kuraridine’s subcellular localization is crucial for its activity and function. It is directed to specific compartments or organelles through targeting signals and post-translational modifications . The localization of Kuraridine within cells can impact its ability to interact with target enzymes and proteins, thereby modulating its biochemical and cellular effects .
Preparation Methods
Synthetic Routes and Reaction Conditions: The synthesis of KURAIDIN involves multiple steps, including the formation of carbon-nitrogen bonds and the introduction of aromatic hydroxyl groups. Transition-metal-catalyzed reactions are often employed to achieve these transformations. For example, catalytic guanylation reactions of amines with carbodiimides and tandem catalytic guanylation/cyclization reactions are commonly used .
Industrial Production Methods: In industrial settings, the production of KURAIDIN may involve large-scale reactions using similar catalytic processes. The use of electrosynthesis methods has also been explored for the preparation of amides, which are structurally related to KURAIDIN . These methods offer advantages in terms of sustainability and safety by generating hazardous reagents in situ rather than handling them directly.
Chemical Reactions Analysis
Types of Reactions: KURAIDIN undergoes various chemical reactions, including oxidation, reduction, and substitution reactions. These reactions are often facilitated by specific reagents and conditions.
Common Reagents and Conditions:
Oxidation: Common oxidizing agents such as potassium permanganate or chromium trioxide can be used to oxidize KURAIDIN.
Reduction: Reducing agents like lithium aluminum hydride or sodium borohydride are employed to reduce KURAIDIN.
Substitution: Substitution reactions may involve reagents such as halogens or nucleophiles under appropriate conditions.
Major Products Formed: The major products formed from these reactions depend on the specific reagents and conditions used. For example, oxidation of KURAIDIN may yield ketones or carboxylic acids, while reduction may produce alcohols or amines.
Scientific Research Applications
KURAIDIN has a wide range of applications in scientific research, including:
Chemistry: KURAIDIN is used as a building block in organic synthesis and as a reagent in various chemical reactions.
Biology: The compound has been studied for its potential biological activities, including its effects on cellular processes and enzyme inhibition.
Medicine: KURAIDIN is being investigated for its therapeutic potential in treating various diseases, including cancer and neurological disorders.
Industry: The compound is used in the production of pharmaceuticals, agrochemicals, and other industrial products.
Comparison with Similar Compounds
KURAIDIN can be compared with other similar compounds based on its chemical structure and properties. Some similar compounds include:
Curcumin: A polyphenolic compound found in turmeric with antioxidant and anti-inflammatory properties
Metformin: A widely used antidiabetic drug with effects on cellular metabolism and neuroprotection.
Uniqueness of KURAIDIN: KURAIDIN is unique due to its specific chemical structure, which includes multiple aromatic hydroxyl groups and a ketone functionality. This structure imparts distinct chemical reactivity and biological activity, making KURAIDIN a valuable compound for various applications.
Properties
CAS No. |
34981-25-4 |
---|---|
Molecular Formula |
C26H30O6 |
Molecular Weight |
438.5 g/mol |
IUPAC Name |
(E)-1-[2,4-dihydroxy-6-methoxy-3-[(2R)-5-methyl-2-prop-1-en-2-ylhex-4-enyl]phenyl]-3-(2,4-dihydroxyphenyl)prop-2-en-1-one |
InChI |
InChI=1S/C26H30O6/c1-15(2)6-7-18(16(3)4)12-20-23(30)14-24(32-5)25(26(20)31)21(28)11-9-17-8-10-19(27)13-22(17)29/h6,8-11,13-14,18,27,29-31H,3,7,12H2,1-2,4-5H3/b11-9+/t18-/m1/s1 |
InChI Key |
PIAPWPAWQGDOMN-SXAWMYDMSA-N |
SMILES |
CC(=CCC(CC1=C(C(=C(C=C1O)OC)C(=O)C=CC2=C(C=C(C=C2)O)O)O)C(=C)C)C |
Isomeric SMILES |
CC(=CC[C@H](CC1=C(C(=C(C=C1O)OC)C(=O)/C=C/C2=C(C=C(C=C2)O)O)O)C(=C)C)C |
Canonical SMILES |
CC(=CCC(CC1=C(C(=C(C=C1O)OC)C(=O)C=CC2=C(C=C(C=C2)O)O)O)C(=C)C)C |
Synonyms |
kuraridin |
Origin of Product |
United States |
Retrosynthesis Analysis
AI-Powered Synthesis Planning: Our tool employs the Template_relevance Pistachio, Template_relevance Bkms_metabolic, Template_relevance Pistachio_ringbreaker, Template_relevance Reaxys, Template_relevance Reaxys_biocatalysis model, leveraging a vast database of chemical reactions to predict feasible synthetic routes.
One-Step Synthesis Focus: Specifically designed for one-step synthesis, it provides concise and direct routes for your target compounds, streamlining the synthesis process.
Accurate Predictions: Utilizing the extensive PISTACHIO, BKMS_METABOLIC, PISTACHIO_RINGBREAKER, REAXYS, REAXYS_BIOCATALYSIS database, our tool offers high-accuracy predictions, reflecting the latest in chemical research and data.
Strategy Settings
Precursor scoring | Relevance Heuristic |
---|---|
Min. plausibility | 0.01 |
Model | Template_relevance |
Template Set | Pistachio/Bkms_metabolic/Pistachio_ringbreaker/Reaxys/Reaxys_biocatalysis |
Top-N result to add to graph | 6 |
Feasible Synthetic Routes
Q1: What is Kuraridine and what is its known biological activity?
A1: Kuraridine is a lavandulylated chalcone found in the Chinese crude drug Sophora flavescens, also known as Ku Shen. [, ] It exhibits antimicrobial activity against Staphylococcus aureus and Streptococcus mutans. [] Interestingly, it is also the first reported chalcone to show glycosidase inhibitory activity, specifically against beta-glucosidase with an IC50 value of 57 μM. []
Q2: How potent is Kuraridine as a glycosidase inhibitor compared to other compounds found in Sophora flavescens?
A2: While Kuraridine shows moderate activity against beta-glucosidase, other lavandulylated flavonoids isolated from Sophora flavescens, such as kushenol A, (-)-kurarinone, and sophoraflavanone G, exhibit stronger inhibitory activities against alpha-glucosidase. [, ] This suggests that the specific structure and substitutions on the flavanone backbone might be crucial for enhancing activity against specific glycosidase enzymes.
Q3: Does the presence of a lavandulyl group influence the biological activity of Kuraridine?
A3: Research suggests that the 8-lavandulyl group in the B-ring of flavonoids significantly contributes to glycosidase inhibitory activities. [] Although Kuraridine is a chalcone and not a flavonoid, it also possesses a lavandulyl group. Further studies are needed to understand the specific role of the lavandulyl group in Kuraridine's interaction with beta-glucosidase.
Q4: Are there any studies investigating the structure-activity relationship of Kuraridine and its analogs?
A4: While the provided research highlights the importance of the lavandulyl group for glycosidase inhibition, [] specific studies focusing on the structure-activity relationship of Kuraridine and its analogs are currently lacking. Investigating how modifications to the Kuraridine scaffold affect its activity, potency, and selectivity towards different glycosidases could be valuable for future drug development.
Disclaimer and Information on In-Vitro Research Products
Please be aware that all articles and product information presented on BenchChem are intended solely for informational purposes. The products available for purchase on BenchChem are specifically designed for in-vitro studies, which are conducted outside of living organisms. In-vitro studies, derived from the Latin term "in glass," involve experiments performed in controlled laboratory settings using cells or tissues. It is important to note that these products are not categorized as medicines or drugs, and they have not received approval from the FDA for the prevention, treatment, or cure of any medical condition, ailment, or disease. We must emphasize that any form of bodily introduction of these products into humans or animals is strictly prohibited by law. It is essential to adhere to these guidelines to ensure compliance with legal and ethical standards in research and experimentation.