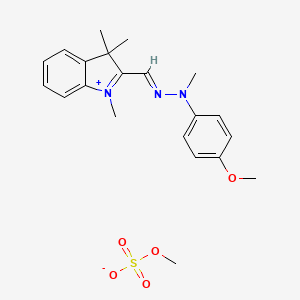
Basic Yellow 28
Overview
Description
Basic Yellow 28: is a cationic dye known for its vibrant yellow color. It is widely used in various applications, including textile dyeing, biological staining, and as a tracer in scientific research. The compound is characterized by its high solubility in water and its ability to bind to negatively charged surfaces, making it useful in a range of industrial and scientific contexts .
Mechanism of Action
Target of Action
Basic Yellow 28, also known as Cationic Yellow X-GL, is primarily used as a dye in various applications . Its primary targets are the materials it is intended to color, such as acrylic fibers, dispersed article fibers, and other types of textiles . It can also be used for direct printing on acrylic and silk, and for dyeing modified polyester, acetate fiber, and PVC fiber .
Mode of Action
The mode of action of this compound involves its interaction with the target material. It is a yellow powder that is soluble in water . When added to a solution with a pH of 6, it interacts with the target material, imparting a yellow color . The dyeing process occurs at 120 °C, and the color remains stable .
Biochemical Pathways
The dye’s interaction with the target material likely involves physical and chemical adsorption processes .
Pharmacokinetics
As a dye, this compound does not have traditional pharmacokinetic properties such as absorption, distribution, metabolism, and excretion (ADME). Instead, its behavior can be described in terms of its solubility, stability, and adsorption characteristics. It is soluble in water and stable at high temperatures . Its adsorption onto target materials is influenced by factors such as temperature, pH, and the presence of other substances .
Result of Action
The primary result of this compound’s action is the imparting of a yellow color to the target material . This is achieved through the dye’s interaction with the material at a molecular level. The dye’s color remains stable even at high temperatures .
Action Environment
The action of this compound is influenced by several environmental factors. The pH of the solution in which the dye is dissolved plays a crucial role in the dyeing process . The temperature also affects the dye’s solubility and its interaction with the target material . Other factors, such as the presence of other substances, can also influence the dye’s action .
Biochemical Analysis
Biochemical Properties
Basic Yellow 28 is known for its interaction with various enzymes, proteins, and other biomolecules. It binds to specific proteins and enzymes, altering their activity and function. For instance, this compound can interact with enzymes involved in metabolic pathways, affecting their catalytic activity. These interactions are often characterized by non-covalent binding, which can lead to changes in the enzyme’s conformation and activity .
Cellular Effects
This compound has significant effects on various types of cells and cellular processes. It influences cell function by affecting cell signaling pathways, gene expression, and cellular metabolism. For example, this compound can alter the expression of genes involved in cell cycle regulation, leading to changes in cell proliferation and differentiation. Additionally, it can impact cellular metabolism by interacting with key metabolic enzymes, thereby affecting the overall metabolic flux within the cell .
Molecular Mechanism
The molecular mechanism of this compound involves its binding interactions with biomolecules, enzyme inhibition or activation, and changes in gene expression. This compound binds to specific sites on enzymes and proteins, leading to conformational changes that either inhibit or activate their function. This binding can also affect the transcription of genes, leading to changes in gene expression patterns. These molecular interactions are crucial for understanding the overall impact of this compound on cellular function .
Temporal Effects in Laboratory Settings
In laboratory settings, the effects of this compound can change over time. The stability and degradation of this compound are important factors that influence its long-term effects on cellular function. Studies have shown that this compound can degrade over time, leading to a decrease in its effectiveness. Additionally, long-term exposure to this compound can result in cumulative effects on cellular function, including changes in gene expression and metabolic activity .
Dosage Effects in Animal Models
The effects of this compound vary with different dosages in animal models. At low doses, this compound may have minimal impact on cellular function, while higher doses can lead to significant changes in cell signaling pathways, gene expression, and metabolic activity. Toxic or adverse effects can also be observed at high doses, including cell death and tissue damage. These dosage-dependent effects are crucial for determining the safe and effective use of this compound in biological experiments .
Metabolic Pathways
This compound is involved in various metabolic pathways, interacting with enzymes and cofactors that regulate metabolic flux. It can affect the levels of metabolites within the cell, leading to changes in overall metabolic activity. For instance, this compound can inhibit key enzymes in glycolysis, leading to a decrease in ATP production and an increase in the accumulation of glycolytic intermediates .
Transport and Distribution
Within cells and tissues, this compound is transported and distributed through specific transporters and binding proteins. These interactions determine the localization and accumulation of this compound within different cellular compartments. For example, this compound can be transported into the nucleus, where it can interact with nuclear proteins and affect gene expression. The distribution of this compound within the cell is crucial for understanding its overall impact on cellular function .
Subcellular Localization
The subcellular localization of this compound is determined by targeting signals and post-translational modifications that direct it to specific compartments or organelles. This compound can localize to the mitochondria, where it can affect mitochondrial function and energy production. Additionally, it can be found in the endoplasmic reticulum, where it can interact with proteins involved in protein folding and secretion. Understanding the subcellular localization of this compound is essential for elucidating its role in cellular function .
Preparation Methods
Synthetic Routes and Reaction Conditions: Basic Yellow 28 is synthesized through a series of chemical reactions involving aromatic amines and sulfonic acids. The process typically involves the following steps:
Diazotization: Aromatic amines are treated with nitrous acid to form diazonium salts.
Coupling Reaction: The diazonium salts are then coupled with sulfonic acid derivatives to form the desired dye.
Purification: The resulting product is purified through crystallization or other separation techniques to obtain this compound in its pure form.
Industrial Production Methods: In industrial settings, the production of this compound involves large-scale chemical reactors where the above reactions are carried out under controlled conditions. The reaction parameters, such as temperature, pH, and concentration, are optimized to maximize yield and purity. The final product is often dried and processed into a powder or liquid form for commercial use .
Chemical Reactions Analysis
Types of Reactions: Basic Yellow 28 undergoes various chemical reactions, including:
Oxidation: The dye can be oxidized under certain conditions, leading to the formation of different colored products.
Reduction: this compound can be reduced to its leuco form, which is colorless.
Substitution: The aromatic rings in this compound can undergo electrophilic substitution reactions.
Common Reagents and Conditions:
Oxidation: Common oxidizing agents include hydrogen peroxide and potassium permanganate.
Reduction: Reducing agents such as sodium dithionite are used.
Substitution: Electrophilic reagents like halogens and nitrating agents are employed.
Major Products Formed:
Oxidation: Various oxidized derivatives with altered color properties.
Reduction: Leuco form of this compound.
Substitution: Substituted aromatic compounds with different functional groups.
Scientific Research Applications
Basic Yellow 28 has a wide range of applications in scientific research:
Biological Staining: It is used to stain cell structures, track biomolecules, and evaluate cell functions in biological experiments.
Environmental Chemistry: The dye is employed in studies related to wastewater treatment and adsorption processes.
Clinical Diagnostics: this compound is used in diagnostic assays to detect and analyze biological molecules.
Textile Industry: It is widely used for dyeing acrylic fibers, modified polyester, acetate fibers, and polyvinyl chloride.
Solar Cells: The dye is used in dye-sensitized solar cells (DSSCs) for its light-absorbing properties.
Comparison with Similar Compounds
Basic Blue 3: Another cationic dye used for similar applications in biological staining and textile dyeing.
Malachite Green: A dye used in biological staining and as an antimicrobial agent.
Tartrazine: A synthetic dye used in food coloring and scientific research.
Uniqueness of Basic Yellow 28: this compound is unique due to its specific color properties and high affinity for negatively charged surfaces. Its versatility in various applications, from biological staining to industrial dyeing, sets it apart from other dyes. Additionally, its ability to function in different pH environments and its stability under various conditions make it a valuable compound in scientific and industrial contexts .
Properties
IUPAC Name |
4-methoxy-N-methyl-N-[(1,3,3-trimethylindol-1-ium-2-yl)methylideneamino]aniline;methyl sulfate | |
---|---|---|
Details | Computed by Lexichem TK 2.7.0 (PubChem release 2021.05.07) | |
Source | PubChem | |
URL | https://pubchem.ncbi.nlm.nih.gov | |
Description | Data deposited in or computed by PubChem | |
InChI |
InChI=1S/C20H24N3O.CH4O4S/c1-20(2)17-8-6-7-9-18(17)22(3)19(20)14-21-23(4)15-10-12-16(24-5)13-11-15;1-5-6(2,3)4/h6-14H,1-5H3;1H3,(H,2,3,4)/q+1;/p-1 | |
Details | Computed by InChI 1.0.6 (PubChem release 2021.05.07) | |
Source | PubChem | |
URL | https://pubchem.ncbi.nlm.nih.gov | |
Description | Data deposited in or computed by PubChem | |
InChI Key |
SPWPAFQLIZTXFN-UHFFFAOYSA-M | |
Details | Computed by InChI 1.0.6 (PubChem release 2021.05.07) | |
Source | PubChem | |
URL | https://pubchem.ncbi.nlm.nih.gov | |
Description | Data deposited in or computed by PubChem | |
Canonical SMILES |
CC1(C2=CC=CC=C2[N+](=C1C=NN(C)C3=CC=C(C=C3)OC)C)C.COS(=O)(=O)[O-] | |
Details | Computed by OEChem 2.3.0 (PubChem release 2021.05.07) | |
Source | PubChem | |
URL | https://pubchem.ncbi.nlm.nih.gov | |
Description | Data deposited in or computed by PubChem | |
Molecular Formula |
C21H27N3O5S | |
Details | Computed by PubChem 2.1 (PubChem release 2021.05.07) | |
Source | PubChem | |
URL | https://pubchem.ncbi.nlm.nih.gov | |
Description | Data deposited in or computed by PubChem | |
DSSTOX Substance ID |
DTXSID20879816 | |
Record name | C.I. Basic Yellow 28 | |
Source | EPA DSSTox | |
URL | https://comptox.epa.gov/dashboard/DTXSID20879816 | |
Description | DSSTox provides a high quality public chemistry resource for supporting improved predictive toxicology. | |
Molecular Weight |
433.5 g/mol | |
Details | Computed by PubChem 2.1 (PubChem release 2021.05.07) | |
Source | PubChem | |
URL | https://pubchem.ncbi.nlm.nih.gov | |
Description | Data deposited in or computed by PubChem | |
CAS No. |
54060-92-3 | |
Record name | Basic Yellow 28 | |
Source | CAS Common Chemistry | |
URL | https://commonchemistry.cas.org/detail?cas_rn=54060-92-3 | |
Description | CAS Common Chemistry is an open community resource for accessing chemical information. Nearly 500,000 chemical substances from CAS REGISTRY cover areas of community interest, including common and frequently regulated chemicals, and those relevant to high school and undergraduate chemistry classes. This chemical information, curated by our expert scientists, is provided in alignment with our mission as a division of the American Chemical Society. | |
Explanation | The data from CAS Common Chemistry is provided under a CC-BY-NC 4.0 license, unless otherwise stated. | |
Record name | C.I. Basic Yellow 28 | |
Source | EPA DSSTox | |
URL | https://comptox.epa.gov/dashboard/DTXSID20879816 | |
Description | DSSTox provides a high quality public chemistry resource for supporting improved predictive toxicology. | |
Retrosynthesis Analysis
AI-Powered Synthesis Planning: Our tool employs the Template_relevance Pistachio, Template_relevance Bkms_metabolic, Template_relevance Pistachio_ringbreaker, Template_relevance Reaxys, Template_relevance Reaxys_biocatalysis model, leveraging a vast database of chemical reactions to predict feasible synthetic routes.
One-Step Synthesis Focus: Specifically designed for one-step synthesis, it provides concise and direct routes for your target compounds, streamlining the synthesis process.
Accurate Predictions: Utilizing the extensive PISTACHIO, BKMS_METABOLIC, PISTACHIO_RINGBREAKER, REAXYS, REAXYS_BIOCATALYSIS database, our tool offers high-accuracy predictions, reflecting the latest in chemical research and data.
Strategy Settings
Precursor scoring | Relevance Heuristic |
---|---|
Min. plausibility | 0.01 |
Model | Template_relevance |
Template Set | Pistachio/Bkms_metabolic/Pistachio_ringbreaker/Reaxys/Reaxys_biocatalysis |
Top-N result to add to graph | 6 |
Feasible Synthetic Routes
ANone: Basic Yellow 28 is a cationic dye, meaning it carries a positive charge. This makes it difficult to remove from wastewater using traditional methods, as it tends to repel negatively charged surfaces found in many natural materials. []
ANone: Several low-cost adsorbents have been investigated for their ability to remove this compound from aqueous solutions. Some examples include:
- Conch shells: Research has shown that conch shells can effectively adsorb this compound from wastewater, with removal efficiencies exceeding 95% under optimal conditions. []
- Activated carbon derived from apricot stones (ASAC): ASAC has demonstrated significant potential for removing this compound, with pH playing a crucial role in adsorption efficiency. []
- Black cumin seed pulp and biochar: Both black cumin seed pulp and its biochar have shown high adsorption capacities for this compound, making them promising candidates for wastewater treatment. []
- Recycled newspaper pulp (NPP): Deinked and bleached NPP can adsorb significant amounts of this compound, offering a potential use for this waste material. []
- Silybum Marianum Stem: Both the natural stem and the carbonized version of Silybum Marianum Stem have shown promising results in removing this compound and Basic Blue 3. []
ANone: The pH of the solution plays a significant role in the adsorption process. For instance, the removal of this compound using activated carbon derived from apricot stones was found to be most effective at a pH of 10. [] Similarly, pH significantly influences the adsorption of this compound onto reed, with an optimal pH of 6 identified for maximum dye uptake. []
ANone: The adsorption kinetics of this compound often align with the pseudo-second-order model. This model suggests that the rate-limiting step in the adsorption process is chemical sorption, involving the formation of chemical bonds between the dye molecules and the adsorbent surface. [, ]
ANone: Yes, photocatalytic degradation, particularly using titanium dioxide (TiO2) as a photocatalyst under UV or visible light irradiation, has proven effective in degrading this compound. [, , , , ]
ANone: Several factors can affect the efficiency of photocatalytic degradation, including:
- Type and intensity of light source: UV-A light is commonly used, and the degradation rate generally increases with increasing light intensity. []
- Concentration of TiO2 catalyst: An optimal concentration of TiO2 exists for maximum degradation efficiency. []
- Initial dye concentration: The degradation rate typically decreases as the initial dye concentration increases. []
- pH of the solution: The pH can influence the surface charge of the TiO2 catalyst and the dye molecule, thereby affecting adsorption and degradation rates. []
- Presence of other ions: The presence of salts, such as NaCl and Na2CO3, can impact the degradation rate. For instance, carbonate ions have been found to enhance the photodegradation rate, while chloride ions can decrease it. []
ANone: Yes, electrochemical techniques, such as electrocoagulation and electrooxidation, have been explored for this compound removal. These methods involve using electrodes to generate reactive species that can degrade the dye molecules. [, , ]
ANone: Different electrode materials exhibit varying efficiencies in degrading this compound. For instance, iron electrodes have shown promising results in electrocoagulation studies. [] In electrooxidation, copper and iron electrodes exhibited higher color and COD removal compared to diamond and aluminum electrodes when treating a solution containing this compound. []
ANone: this compound, like many synthetic dyes, can have toxicological effects on aquatic organisms. Studies using Drosophila melanogaster have shown that exposure to this compound can affect lifespan, survival rate, and reproductive success. []
ANone: this compound is known to have limited biodegradability, meaning it can persist in the environment for extended periods. This persistence raises concerns about its potential long-term impact on ecosystems. []
ANone: Coupling electrochemical pretreatment with biological treatment has shown promise in enhancing the biodegradability of this compound. The electrochemical process can break down the dye molecules into smaller, more biodegradable byproducts, making it easier for microorganisms to further degrade them in a subsequent biological treatment stage. [, ]
ANone: Various analytical methods are employed to monitor the degradation process, including:
- UV-Vis spectrophotometry: This technique is widely used to measure the absorbance of the dye solution at specific wavelengths, allowing for the quantification of dye concentration changes during treatment. [, ]
- Chemical Oxygen Demand (COD) analysis: COD measurements provide an indication of the organic load in the solution, helping assess the extent of dye degradation. [, ]
- Total Organic Carbon (TOC) analysis: TOC analysis measures the total amount of carbon in organic compounds, offering a comprehensive assessment of the mineralization of the dye during treatment. [, ]
- High-Performance Liquid Chromatography (HPLC): HPLC can be coupled with detectors like diode array detectors to identify and quantify the dye and its degradation products, providing insights into the degradation pathway. []
Disclaimer and Information on In-Vitro Research Products
Please be aware that all articles and product information presented on BenchChem are intended solely for informational purposes. The products available for purchase on BenchChem are specifically designed for in-vitro studies, which are conducted outside of living organisms. In-vitro studies, derived from the Latin term "in glass," involve experiments performed in controlled laboratory settings using cells or tissues. It is important to note that these products are not categorized as medicines or drugs, and they have not received approval from the FDA for the prevention, treatment, or cure of any medical condition, ailment, or disease. We must emphasize that any form of bodily introduction of these products into humans or animals is strictly prohibited by law. It is essential to adhere to these guidelines to ensure compliance with legal and ethical standards in research and experimentation.