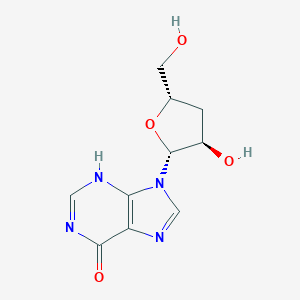
3'-Deoxyinosine
Overview
Description
Mechanism of Action
Target of Action
3’-Deoxyinosine, a purine nucleoside analog, primarily targets indolent lymphoid malignancies . It interacts with the DNA repair enzyme, DNA-deoxyinosine glycosylase , which is involved in DNA damage repair and targets hypoxanthine bases .
Mode of Action
The mode of action of 3’-Deoxyinosine involves inhibiting DNA synthesis and inducing apoptosis . It is recognized by the DNA repair enzyme Endonuclease V (endo V), which was first discovered as a deoxyinosine 3′ endonuclease . Endo V plays a crucial role as a DNA repair enzyme for the removal of deaminated bases .
Biochemical Pathways
The biochemical pathways affected by 3’-Deoxyinosine are related to DNA repair. Deaminated lesions in DNA bases are removed through the base excision repair (BER) pathway . This pathway is initiated by DNA glycosylase to remove a mismatch or mutated base . The DNA-deoxyinosine glycosylase activity, the removal of a hypoxanthine base, is observed within the uracil DNA glycosylase superfamily .
Result of Action
The result of 3’-Deoxyinosine’s action is the inhibition of tumor growth. It has broad antitumor activity, particularly against indolent lymphoid malignancies . The anticancer mechanisms in this process rely on the inhibition of DNA synthesis and the induction of apoptosis .
Action Environment
The action of 3’-Deoxyinosine can be influenced by various environmental factors. For instance, the presence of certain medicinal plants can enhance the production of cordycepin, a major active ingredient of Cordyceps militaris, which is also known as 3’-deoxyadenosine . This suggests that the environment can play a significant role in the efficacy and stability of 3’-Deoxyinosine.
Biochemical Analysis
Biochemical Properties
3’-Deoxyinosine interacts with various enzymes and proteins. It is recognized by endonuclease V (endo V), which was first discovered in Escherichia coli and later rediscovered as a deoxyinosine 3′ endonuclease . Endo V plays a crucial role as a DNA repair enzyme for the removal of deaminated bases .
Cellular Effects
3’-Deoxyinosine can arise from spontaneous deamination of deoxyadenosine residue, and is also induced by ROS produced from normal aerobic respiration . In addition, exposure of DNA to ionizing radiation, UV light, nitrous acid, or heat can promote the formation of 3’-Deoxyinosine . It is potentially mutagenic since it prefers to pair with dCTP during replication, yielding A:T to G:C transition mutations at sites of adenine deamination .
Molecular Mechanism
The molecular mechanism of 3’-Deoxyinosine involves the conversion of the 6-aminopurine ring of adenosine to a 6-oxopurine ring. This process is carried out by specific RNA deaminases . In addition, 3’-Deoxyinosine can be converted to cordycepin 5′-triphosphate, the active metabolite of cordycepin .
Temporal Effects in Laboratory Settings
The thermal stability of oligodeoxyribonucleotide duplexes containing 3’-Deoxyinosine residues has been studied using optical melting techniques . The stability of these duplexes can change over time, affecting the effects of 3’-Deoxyinosine on cellular function .
Dosage Effects in Animal Models
The selection of appropriate animal models is critical, considering factors such as physiological and pathophysiological similarities, availability, and ethical considerations .
Metabolic Pathways
3’-Deoxyinosine is involved in purine metabolism . It serves as a key intermediate in purine metabolism and is a widespread component of various biochemical processes .
Subcellular Localization
Mrna localization to distinct cellular compartments gives precise and efficient control over the translation process . This suggests that 3’-Deoxyinosine, as a component of mRNA, may also be subject to similar localization mechanisms .
Preparation Methods
Synthetic Routes and Reaction Conditions: 3’-Deoxyinosine can be synthesized through the deacetylation of diacetate precursors. For instance, a solution of diacetate in methanol is treated with ammonium hydroxide at room temperature for about an hour . This reaction yields 3’-Deoxyinosine after the removal of acetyl groups.
Industrial Production Methods: While specific industrial production methods for 3’-Deoxyinosine are not extensively documented, the general approach involves large-scale synthesis using similar deacetylation reactions under controlled conditions to ensure high purity and yield.
Chemical Reactions Analysis
Types of Reactions: 3’-Deoxyinosine undergoes various chemical reactions, including:
Oxidation: This reaction can convert 3’-Deoxyinosine into other oxidized forms.
Reduction: Reduction reactions can modify the purine ring structure.
Substitution: Substitution reactions can introduce different functional groups at specific positions on the molecule.
Common Reagents and Conditions:
Oxidation: Common oxidizing agents include hydrogen peroxide and potassium permanganate.
Reduction: Reducing agents such as sodium borohydride are often used.
Substitution: Conditions vary depending on the desired substitution, but typical reagents include halogenating agents and nucleophiles.
Major Products: The major products formed from these reactions depend on the specific conditions and reagents used. For example, oxidation may yield various oxidized purine derivatives, while substitution can introduce new functional groups, altering the compound’s properties.
Scientific Research Applications
3’-Deoxyinosine has a wide range of applications in scientific research:
Chemistry: It is used as a building block in the synthesis of more complex molecules.
Biology: It plays a role in studying DNA repair mechanisms and mutagenesis.
Comparison with Similar Compounds
Cordycepin (3’-Deoxyadenosine): Similar in structure but with an adenine base instead of hypoxanthine.
Deoxyadenosine: Lacks the hydroxy group at the 2’ position but has an adenine base.
Deoxyguanosine: Similar structure with a guanine base.
Uniqueness: 3’-Deoxyinosine is unique due to its specific interactions with DNA and its ability to induce mutations, making it a valuable tool in genetic research and cancer treatment .
By understanding the properties and applications of 3’-Deoxyinosine, researchers can leverage its potential in various scientific and industrial fields.
Properties
IUPAC Name |
9-[(2R,3R,5S)-3-hydroxy-5-(hydroxymethyl)oxolan-2-yl]-1H-purin-6-one | |
---|---|---|
Source | PubChem | |
URL | https://pubchem.ncbi.nlm.nih.gov | |
Description | Data deposited in or computed by PubChem | |
InChI |
InChI=1S/C10H12N4O4/c15-2-5-1-6(16)10(18-5)14-4-13-7-8(14)11-3-12-9(7)17/h3-6,10,15-16H,1-2H2,(H,11,12,17)/t5-,6+,10+/m0/s1 | |
Source | PubChem | |
URL | https://pubchem.ncbi.nlm.nih.gov | |
Description | Data deposited in or computed by PubChem | |
InChI Key |
RPZDLTVHZJHPAW-BAJZRUMYSA-N | |
Source | PubChem | |
URL | https://pubchem.ncbi.nlm.nih.gov | |
Description | Data deposited in or computed by PubChem | |
Canonical SMILES |
C1C(OC(C1O)N2C=NC3=C2N=CNC3=O)CO | |
Source | PubChem | |
URL | https://pubchem.ncbi.nlm.nih.gov | |
Description | Data deposited in or computed by PubChem | |
Isomeric SMILES |
C1[C@H](O[C@H]([C@@H]1O)N2C=NC3=C2N=CNC3=O)CO | |
Source | PubChem | |
URL | https://pubchem.ncbi.nlm.nih.gov | |
Description | Data deposited in or computed by PubChem | |
Molecular Formula |
C10H12N4O4 | |
Source | PubChem | |
URL | https://pubchem.ncbi.nlm.nih.gov | |
Description | Data deposited in or computed by PubChem | |
DSSTOX Substance ID |
DTXSID70927210 | |
Record name | 9-(3-Deoxypentofuranosyl)-9H-purin-6-ol | |
Source | EPA DSSTox | |
URL | https://comptox.epa.gov/dashboard/DTXSID70927210 | |
Description | DSSTox provides a high quality public chemistry resource for supporting improved predictive toxicology. | |
Molecular Weight |
252.23 g/mol | |
Source | PubChem | |
URL | https://pubchem.ncbi.nlm.nih.gov | |
Description | Data deposited in or computed by PubChem | |
CAS No. |
13146-72-0 | |
Record name | 3'-Deoxyinosine | |
Source | ChemIDplus | |
URL | https://pubchem.ncbi.nlm.nih.gov/substance/?source=chemidplus&sourceid=0013146720 | |
Description | ChemIDplus is a free, web search system that provides access to the structure and nomenclature authority files used for the identification of chemical substances cited in National Library of Medicine (NLM) databases, including the TOXNET system. | |
Record name | 9-(3-Deoxypentofuranosyl)-9H-purin-6-ol | |
Source | EPA DSSTox | |
URL | https://comptox.epa.gov/dashboard/DTXSID70927210 | |
Description | DSSTox provides a high quality public chemistry resource for supporting improved predictive toxicology. | |
Record name | 3'-DEOXYINOSINE | |
Source | FDA Global Substance Registration System (GSRS) | |
URL | https://gsrs.ncats.nih.gov/ginas/app/beta/substances/J71U8QAU3Q | |
Description | The FDA Global Substance Registration System (GSRS) enables the efficient and accurate exchange of information on what substances are in regulated products. Instead of relying on names, which vary across regulatory domains, countries, and regions, the GSRS knowledge base makes it possible for substances to be defined by standardized, scientific descriptions. | |
Explanation | Unless otherwise noted, the contents of the FDA website (www.fda.gov), both text and graphics, are not copyrighted. They are in the public domain and may be republished, reprinted and otherwise used freely by anyone without the need to obtain permission from FDA. Credit to the U.S. Food and Drug Administration as the source is appreciated but not required. | |
Retrosynthesis Analysis
AI-Powered Synthesis Planning: Our tool employs the Template_relevance Pistachio, Template_relevance Bkms_metabolic, Template_relevance Pistachio_ringbreaker, Template_relevance Reaxys, Template_relevance Reaxys_biocatalysis model, leveraging a vast database of chemical reactions to predict feasible synthetic routes.
One-Step Synthesis Focus: Specifically designed for one-step synthesis, it provides concise and direct routes for your target compounds, streamlining the synthesis process.
Accurate Predictions: Utilizing the extensive PISTACHIO, BKMS_METABOLIC, PISTACHIO_RINGBREAKER, REAXYS, REAXYS_BIOCATALYSIS database, our tool offers high-accuracy predictions, reflecting the latest in chemical research and data.
Strategy Settings
Precursor scoring | Relevance Heuristic |
---|---|
Min. plausibility | 0.01 |
Model | Template_relevance |
Template Set | Pistachio/Bkms_metabolic/Pistachio_ringbreaker/Reaxys/Reaxys_biocatalysis |
Top-N result to add to graph | 6 |
Feasible Synthetic Routes
Q1: How does 3'-deoxyinosine exert its anti-parasitic effects?
A1: this compound exhibits potent anti-parasitic activity, particularly against Leishmania species. It is taken up by the parasite and metabolized into this compound-5'-monophosphate. The parasite further converts this into 3'-deoxyadenosine (cordycepin)-5'-mono, di-, and triphosphates. These phosphorylated forms are highly toxic and interfere with crucial cellular processes, ultimately leading to parasite death. []
Q2: Does this compound affect mammalian cells?
A2: While this compound shows potent anti-parasitic activity, it exhibits significantly lower toxicity towards mammalian cells. This selectivity makes it a promising candidate for further development as an anti-parasitic agent. [] For instance, it is remarkably less toxic towards mouse mammary tumor FM3A cells compared to Leishmania tropica promastigotes. []
Q3: How does adenosine deaminase (ADA) impact 3'-deoxyadenosine (cordycepin) activity?
A3: ADA plays a crucial role in the metabolism of cordycepin (3'-deoxyadenosine). It converts cordycepin into the less active metabolite this compound, thus reducing its efficacy. [, , , ] Therefore, combining cordycepin with ADA inhibitors could be a promising strategy for cancer treatment. []
Q4: Can you explain the impact of ADA on the therapeutic potential of cordycepin?
A4: ADA expression levels in tumor cells can be used as a predictive biomarker for cordycepin's effectiveness. Tumor cells with low ADA activity are more sensitive to cordycepin treatment. [] This suggests that ADA inhibitors, in combination with cordycepin, might be a valuable strategy to treat a broader range of cancers, particularly those with high ADA activity. []
Q5: What is the molecular formula and weight of this compound?
A5: The molecular formula of this compound is C10H12N4O4, and its molecular weight is 252.23 g/mol.
Q6: How do structural modifications of inosine affect its hypotensive activity?
A6: The ribose configuration of inosine plays a critical role in its hypotensive activity. Modifications at the 2' and 3' positions of the ribose moiety, such as in 2'-deoxyinosine and this compound, result in a complete loss of hypotensive effects. []
Q7: What is known about the pharmacokinetics of cordycepin?
A7: Cordycepin is known to undergo rapid deamination in vivo, primarily by ADA, forming this compound. This conversion can significantly impact its pharmacokinetic profile. [] To obtain accurate pharmacokinetic data, it is crucial to prevent this degradation during blood sample collection, such as by adding methanol. []
Q8: How does the ADA inhibitor erythro-9-(2-hydroxy-3-nonyl) adenine hydrochloride influence cordycepin's pharmacokinetics?
A8: Erythro-9-(2-hydroxy-3-nonyl) adenine hydrochloride, a known ADA inhibitor, can significantly prolong the half-life of cordycepin in vivo. This highlights the potential of ADA inhibitors in improving the pharmacokinetic profile and therapeutic efficacy of cordycepin. []
Q9: Is this compound effective against Leishmania donovani, and how does its efficacy compare to other inosine analogs?
A9: Yes, this compound demonstrates significant anti-leishmanial activity against Leishmania donovani amastigotes in vitro and in vivo. [] In a study using infected mice, intravenous administration of this compound at 100 mg/kg resulted in a 68% reduction in parasite load, compared to untreated controls. [] Among the tested inosine analogs, carbocyclic inosine (C-Ino) exhibited the most potent inhibitory effect, followed by this compound (3'-dI) and 3'-fluoroinosine (3'-FI). []
Q10: Can liposomal encapsulation enhance the efficacy of inosine analogs against leishmaniasis?
A10: Yes, encapsulating inosine analogs in liposomes can significantly enhance their therapeutic efficacy against Leishmania donovani infection. [] Liposomal formulations of C-Ino, 3'-dI, and 3'-FI, administered at 10 mg/kg, achieved comparable or even greater reductions in parasite load compared to their non-encapsulated counterparts at significantly higher doses (50-100 mg/kg). [] This suggests that liposomal delivery can potentially reduce the required dosage and minimize potential side effects. []
Q11: How can researchers screen for potential ADA inhibitors from natural sources?
A11: A rapid and sensitive method using ultra-high performance liquid chromatography-tandem mass spectrometry (UHPLC-MS/MS) can be employed to screen for ADA inhibitors from natural sources. [] This method directly measures the conversion of cordycepin to this compound in the presence of potential inhibitors. By quantifying this compound levels, researchers can evaluate the ADA inhibitory activity of test compounds. [] For instance, this method identified oleanolic acid and ursolic acid from Ligustri lucidi fructus as potent ADA inhibitors. []
Q12: Are there other potential therapeutic applications for compounds isolated from Cordyceps militaris?
A12: Yes, besides this compound, another compound isolated from Cordyceps militaris-inoculated Bombyx mori is cordycepin. [] Cordycepin exhibits cytotoxic activity against various cancer cell lines, including A549 (lung cancer), PANC-1 (pancreatic cancer), and MCF-7 (breast cancer). [] This suggests that compounds derived from Cordyceps militaris hold potential for developing novel anticancer therapies. []
Disclaimer and Information on In-Vitro Research Products
Please be aware that all articles and product information presented on BenchChem are intended solely for informational purposes. The products available for purchase on BenchChem are specifically designed for in-vitro studies, which are conducted outside of living organisms. In-vitro studies, derived from the Latin term "in glass," involve experiments performed in controlled laboratory settings using cells or tissues. It is important to note that these products are not categorized as medicines or drugs, and they have not received approval from the FDA for the prevention, treatment, or cure of any medical condition, ailment, or disease. We must emphasize that any form of bodily introduction of these products into humans or animals is strictly prohibited by law. It is essential to adhere to these guidelines to ensure compliance with legal and ethical standards in research and experimentation.