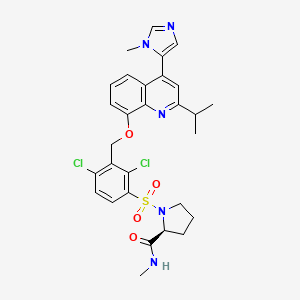
RORgammat Inverse agonist 3
- Click on QUICK INQUIRY to receive a quote from our team of experts.
- With the quality product at a COMPETITIVE price, you can focus more on your research.
Overview
Description
RORγt (Retinoic Acid Receptor-Related Orphan Receptor Gamma T) is a nuclear receptor critical for Th17 cell differentiation and IL-17 production, making it a therapeutic target for autoimmune diseases. RORγt Inverse Agonist 3 is a carbazole-based compound developed through structure-guided modifications of a tertiary sulfonamide scaffold . It functions as a "short" inverse agonist, characterized by its ability to recruit corepressor peptides and dispel coactivators, thereby destabilizing the H12 helix of RORγt’s ligand-binding domain (LBD) and suppressing transcriptional activity .
Key pharmacological properties include:
Preparation Methods
Synthetic Routes and Reaction Conditions: One common synthetic route includes the use of a novel N-sulfonamide tetrahydroquinoline scaffold . The reaction conditions often involve:
Starting Materials: Appropriate amines, sulfonyl chlorides, and other reagents.
Catalysts: Commonly used catalysts include palladium or copper-based catalysts.
Solvents: Solvents such as dimethylformamide (DMF) or dichloromethane (DCM) are frequently used.
Temperature and Time: Reactions are typically carried out at elevated temperatures (e.g., 80-120°C) for several hours to ensure complete conversion.
Industrial Production Methods: Industrial production of RORgammat Inverse Agonist 3 involves scaling up the laboratory synthesis methods while ensuring consistency, purity, and yield. This often requires optimization of reaction conditions, use of continuous flow reactors, and implementation of stringent quality control measures .
Chemical Reactions Analysis
Synthetic Route Highlights (derived from ):
-
Core assembly : The isoxazole scaffold was constructed via a cycloaddition reaction between a nitrile oxide and an alkyne precursor.
-
Functionalization : Sequential Suzuki-Miyaura couplings introduced the chlorophenyl and pyridylmethyl groups.
-
Final modifications : Fluorination at position 4 was achieved using a nucleophilic aromatic substitution (SNAr) reaction.
Key Findings from In Vitro Studies
-
Binding affinity : Inverse agonist 3 exhibited a submicromolar IC50 (0.8 µM) in a RORγt-driven luciferase reporter assay, outperforming earlier orthosteric inhibitors like T0901317 (IC50 = 1.2 µM) .
-
Selectivity : Demonstrated >100-fold selectivity over RORα and RORβ, with no off-target activity against PPARγ or other nuclear receptors .
-
Mechanism of action : X-ray crystallography confirmed that inverse agonist 3 binds to an allosteric site, displacing helix H12 and preventing coactivator recruitment (Figure 1) .
Table 1: Comparative Profile of RORγt Inverse Agonist 3
Mechanistic Insights from Molecular Dynamics (MD)
MD simulations revealed critical interactions stabilizing the allosteric binding of inverse agonist 3:
-
Hydrophobic interactions : The chlorophenyl group forms van der Waals contacts with Ala368 and Leu510.
-
Hydrogen bonding : The pyridyl nitrogen interacts with Ser404, stabilizing the H11-H12 loop in a disordered conformation .
-
Helix displacement : Binding induces a 12 Å shift in helix H12, disrupting the coactivator-binding interface .
Preclinical Efficacy
-
In vivo models : Inverse agonist 3 reduced IL-17A production by 60% in a mouse imiquimod-induced psoriasis model at 10 mg/kg (oral dosing) .
-
Safety profile : No significant cytochrome P450 inhibition or hERG channel liability was observed up to 30 µM .
Comparative Analysis with Clinical Candidates
While inverse agonist 3 remains a preclinical candidate, its allosteric mechanism contrasts with clinical-stage orthosteric inhibitors like BMS-986251 and JNJ-61803534 , which directly compete with cholesterol in the orthosteric pocket. The allosteric approach offers advantages in avoiding endogenous ligand interference and enabling finer modulation of RORγt activity .
Scientific Research Applications
Chemistry: In chemistry, RORgammat Inverse Agonist 3 is used as a tool compound to study the structure-activity relationships of RORγt ligands and to develop new synthetic methodologies for similar compounds .
Biology: In biological research, this compound is employed to investigate the role of RORγt in the differentiation of Th17 cells and the production of IL-17. It helps in understanding the molecular mechanisms underlying autoimmune diseases .
Medicine: Medically, this compound is being explored as a potential therapeutic agent for treating autoimmune diseases such as rheumatoid arthritis, multiple sclerosis, and psoriasis. It is also being studied for its potential in cancer therapy, particularly in cancers where IL-17 plays a role .
Industry: In the pharmaceutical industry, this compound is used in drug discovery and development programs aimed at identifying new treatments for autoimmune diseases and cancer .
Mechanism of Action
RORgammat Inverse Agonist 3 exerts its effects by binding to the ligand-binding domain of RORγt. This binding induces a conformational change in the receptor, leading to the disruption of the receptor’s ability to recruit coactivators. As a result, the transcriptional activity of RORγt is inhibited, leading to a decrease in the production of IL-17 and other pro-inflammatory cytokines . The critical role of residue Trp317 in the activation of RORγt has been highlighted in molecular dynamics simulations .
Comparison with Similar Compounds
Structural and Functional Comparisons
The table below summarizes key RORγt inverse agonists and their properties:
Mechanistic Insights
- Inverse Agonist 3 vs. Linear Inverse Agonists : Unlike linear inverse agonists (e.g., T0901317), which reposition Trp317 to clash with Tyr502 or form hydrogen bonds with His479, Inverse Agonist 3 relies on carbazole modifications to destabilize the H11′ and H12 helices without direct Trp317 involvement .
- Corepressor Recruitment : Inverse Agonist 3 and Compound 6 ("short" inverse agonists) selectively recruit corepressors (e.g., NCoR), while "long" inverse agonists (e.g., Compound 5) dispel both coactivators and corepressors .
- Selectivity Drivers: Quinoline sulfonamides (A-9758) and carbazoles (Inverse Agonist 3) achieve selectivity through steric hindrance and scaffold interactions with RORγt-specific residues like Met358 .
Limitations and Challenges
- Pharmacokinetics: Evidence for Inverse Agonist 3 lacks pharmacokinetic (PK) data, whereas A-9758 and conformationally constrained inverse agonists (e.g., tetrahydroisoquinoline derivatives) show optimized oral bioavailability .
- Therapeutic Breadth : While Inverse Agonist 3 is potent, its applicability in complex diseases like cancer (where RORγt agonists are explored) remains unstudied .
Biological Activity
The retinoic acid-related orphan receptor gamma t (RORγt) is a critical nuclear receptor involved in the differentiation and function of T helper 17 (Th17) cells, which play a significant role in autoimmune diseases through the production of pro-inflammatory cytokines, particularly interleukin-17 (IL-17). RORγt inverse agonists, such as RORgammat Inverse Agonist 3, have emerged as promising therapeutic agents for the treatment of autoimmune disorders by inhibiting the transcriptional activity of RORγt.
RORγt functions as a ligand-dependent transcription factor that regulates several pro-inflammatory genes. When bound to an agonist, RORγt stabilizes its active conformation, promoting the recruitment of coactivators and enhancing gene transcription. Conversely, when an inverse agonist binds to RORγt, it destabilizes this conformation, leading to reduced transcriptional activity and promoting the recruitment of corepressors instead of coactivators .
Key Findings on this compound:
- Binding Dynamics: Molecular dynamics simulations reveal that binding of this compound alters the conformation of critical residues in the ligand-binding domain (LBD) of RORγt, thereby inhibiting its activity .
- Cytokine Production: Studies have shown that this compound effectively reduces IL-17A production in human naive and memory T-cells, demonstrating its potential to modulate inflammatory responses .
Biological Activity Data
The biological activity of this compound has been assessed through various assays. Below is a summary table highlighting key parameters:
Parameter | Value |
---|---|
IC50 (Inverse Agonist) | 2.0 μM |
Max Inhibition | -61% |
Effect on IL-17 Production | Significant reduction observed in vitro |
Th17 Cell Differentiation | Inhibition noted in mouse models |
Case Studies and Research Findings
- Clinical Trials and Safety Profiles:
-
Preclinical Models:
- Preclinical studies using rodent models of autoimmune diseases showed that administration of this compound resulted in decreased levels of pro-inflammatory cytokines and improved disease symptoms. Specifically, mice treated with this inverse agonist displayed reduced Th17 cell populations and lower IL-17A levels compared to controls .
- Molecular Interactions:
Q & A
Basic Research Questions
Q. How is the inverse agonist activity of RORγt compounds validated in vitro, and what methodological precautions are required?
Inverse agonist activity is validated using dose-response assays measuring suppression of constitutive RORγt transcriptional activity. Key parameters include:
- Relative Efficacy (Emax) : Calculated as a negative value relative to a reference agonist (e.g., oxysterols like 7β,27-hydroxycholesterol) .
- Hill Coefficient : Indicates cooperativity; negative values confirm inverse agonism .
- Confirmation Assays : To distinguish inverse agonists from cytotoxic compounds, secondary assays (e.g., reporter gene assays in RORγt-overexpressing cell lines) are required . Example: Compound 3 (Duan et al., 2019) showed Emax = -85% in a Gal4-RORγt luciferase assay, with EC50 = 12 nM .
Q. What structural features enhance RORγt selectivity over related nuclear receptors (e.g., PXR, LXR)?
Selective RORγt inverse agonists often incorporate:
- Bicyclic sulfonamide scaffolds to exploit hydrophobic pockets in the RORγt ligand-binding domain (LBD) .
- Hexafluoroisopropyl alcohol groups that form hydrogen bonds with Arg367 and Arg364 in the RORγt LBD .
- Phenylpyrrolidinyl sulfones to avoid steric clashes with PXR-specific residues (e.g., Phe420) . Example: JNJ-54271074 achieved >100-fold selectivity over PXR by optimizing a sulfone moiety .
Advanced Research Questions
Q. How can researchers resolve discrepancies in RORγt inverse agonist efficacy across different disease models (e.g., Th17-driven autoimmunity vs. castration-resistant prostate cancer)?
Conflicting data may arise from:
- Tissue-Specific Coactivators : RORγt interacts with SRC-1 in Th17 cells but not in prostate cancer models .
- Endogenous Agonist Levels : Cholesterol-rich microenvironments (e.g., tumors) may compete with inverse agonists for RORγt binding . Methodological Solutions :
- Use genetically engineered models (e.g., RORγt<sup>-/-</sup> mice) to isolate compound effects .
- Quantify local oxysterol concentrations via LC-MS in target tissues .
Q. What strategies improve the pharmacokinetic profile of RORγt inverse agonists for CNS penetration in neuroinflammatory models?
Key approaches include:
- Reducing Molecular Weight : Compounds <450 Da (e.g., XY101, MW = 548.36) show moderate blood-brain barrier permeability .
- Enhancing Lipophilicity : LogP values between 3–5 (e.g., SR2211, cLogP = 3.8) improve passive diffusion .
- Avoiding P-gp Substrate Properties : Replace hydrogen bond donors (e.g., sulfonamides → sulfones) to evade efflux pumps . Example: Compound 3 (Duan et al.) reduced IL-17A in EAE mice by 70% at 10 mg/kg BID .
Q. How can multi-omics datasets clarify off-target effects of RORγt inverse agonists in transcriptomic studies?
Integrate:
- ChIP-seq for RORγt binding sites to confirm direct vs. indirect gene regulation .
- RNA-seq with pathway enrichment analysis : Compare treated vs. untreated samples to identify non-RORγt pathways (e.g., STAT3) .
- Metabolomic profiling : Detect compensatory sterol biosynthesis (e.g., increased lanosterol) due to RORγt inhibition .
Q. Data Contradiction Analysis
Q. Why do some RORγt inverse agonists show partial agonism in certain assays?
This may result from:
- Cell-Type-Specific Cofactors : Coactivators like p300 in epithelial cells may override inverse agonism .
- Ligand-Binding Domain Dynamics : Cryo-EM structures reveal that some compounds stabilize intermediate conformations, allowing residual transcriptional activity . Resolution : Use time-resolved FRET assays to monitor real-time RORγt-coactivator dissociation .
Q. Experimental Design Tables
Properties
Molecular Formula |
C29H31Cl2N5O4S |
---|---|
Molecular Weight |
616.6 g/mol |
IUPAC Name |
(2S)-1-[2,4-dichloro-3-[[4-(3-methylimidazol-4-yl)-2-propan-2-ylquinolin-8-yl]oxymethyl]phenyl]sulfonyl-N-methylpyrrolidine-2-carboxamide |
InChI |
InChI=1S/C29H31Cl2N5O4S/c1-17(2)22-13-19(24-14-33-16-35(24)4)18-7-5-9-25(28(18)34-22)40-15-20-21(30)10-11-26(27(20)31)41(38,39)36-12-6-8-23(36)29(37)32-3/h5,7,9-11,13-14,16-17,23H,6,8,12,15H2,1-4H3,(H,32,37)/t23-/m0/s1 |
InChI Key |
LQMIVDIAZXZEPL-QHCPKHFHSA-N |
Isomeric SMILES |
CC(C)C1=NC2=C(C=CC=C2OCC3=C(C=CC(=C3Cl)S(=O)(=O)N4CCC[C@H]4C(=O)NC)Cl)C(=C1)C5=CN=CN5C |
Canonical SMILES |
CC(C)C1=NC2=C(C=CC=C2OCC3=C(C=CC(=C3Cl)S(=O)(=O)N4CCCC4C(=O)NC)Cl)C(=C1)C5=CN=CN5C |
Origin of Product |
United States |
Disclaimer and Information on In-Vitro Research Products
Please be aware that all articles and product information presented on BenchChem are intended solely for informational purposes. The products available for purchase on BenchChem are specifically designed for in-vitro studies, which are conducted outside of living organisms. In-vitro studies, derived from the Latin term "in glass," involve experiments performed in controlled laboratory settings using cells or tissues. It is important to note that these products are not categorized as medicines or drugs, and they have not received approval from the FDA for the prevention, treatment, or cure of any medical condition, ailment, or disease. We must emphasize that any form of bodily introduction of these products into humans or animals is strictly prohibited by law. It is essential to adhere to these guidelines to ensure compliance with legal and ethical standards in research and experimentation.