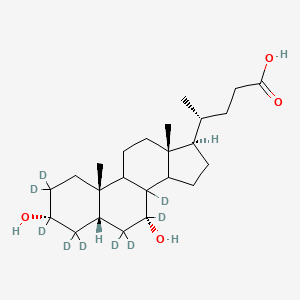
Chenodeoxycholic Acid-d9
- Click on QUICK INQUIRY to receive a quote from our team of experts.
- With the quality product at a COMPETITIVE price, you can focus more on your research.
Overview
Description
Chenodeoxycholic Acid-d9 is a deuterated form of Chenodeoxycholic Acid, a primary bile acid synthesized in the liver from cholesterol. This compound is known for its role in the metabolism of cholesterol and its involvement in various physiological processes. The deuterated form, this compound, is often used in scientific research to study metabolic pathways and the pharmacokinetics of bile acids .
Preparation Methods
Synthetic Routes and Reaction Conditions
Chenodeoxycholic Acid can be synthesized through several chemical routes. One common method involves the extraction of Chenodeoxycholic Acid from bile, followed by chemical synthesis to introduce the deuterium atoms. The synthesis typically involves multiple steps, including hydroxylation and oxidation reactions, under controlled conditions .
Industrial Production Methods
Industrial production of Chenodeoxycholic Acid-d9 involves large-scale extraction from animal bile, followed by chemical modification to introduce deuterium. This process requires precise control of reaction conditions to ensure high yield and purity of the final product .
Chemical Reactions Analysis
Types of Reactions
Chenodeoxycholic Acid-d9 undergoes various chemical reactions, including:
Oxidation: Conversion of hydroxyl groups to ketones.
Reduction: Reduction of ketones back to hydroxyl groups.
Substitution: Replacement of hydrogen atoms with other functional groups.
Common Reagents and Conditions
Common reagents used in these reactions include oxidizing agents like potassium permanganate and reducing agents like sodium borohydride. The reactions are typically carried out under controlled temperatures and pH conditions to ensure specificity and yield .
Major Products
The major products formed from these reactions include various hydroxylated and ketone derivatives of this compound, which are used in further biochemical studies .
Scientific Research Applications
Chenodeoxycholic Acid-d9 has a wide range of applications in scientific research:
Chemistry: Used as a tracer in metabolic studies to understand the pathways of bile acid synthesis and degradation.
Biology: Helps in studying the role of bile acids in cellular signaling and metabolism.
Medicine: Investigated for its potential therapeutic effects in treating cholesterol-related disorders and gallstones.
Industry: Used in the development of pharmaceuticals and as a standard in analytical chemistry
Mechanism of Action
Chenodeoxycholic Acid-d9 exerts its effects by activating nuclear receptors such as the farnesoid X receptor (FXR). This activation leads to the regulation of genes involved in cholesterol metabolism and bile acid synthesis. The compound also inhibits the enzyme 3-hydroxy-3-methylglutaryl coenzyme A reductase, reducing cholesterol synthesis in the liver .
Comparison with Similar Compounds
Similar Compounds
Cholic Acid: Another primary bile acid with similar functions but different hydroxylation patterns.
Ursodeoxycholic Acid: A secondary bile acid used therapeutically for gallstone dissolution.
Deoxycholic Acid: A secondary bile acid involved in fat emulsification.
Uniqueness
Chenodeoxycholic Acid-d9 is unique due to its deuterium labeling, which makes it an invaluable tool in tracing metabolic pathways and studying the pharmacokinetics of bile acids. This labeling provides more precise data in research compared to non-deuterated forms .
Properties
Molecular Formula |
C24H40O4 |
---|---|
Molecular Weight |
401.6 g/mol |
IUPAC Name |
(4R)-4-[(3R,5S,7R,10S,13R,17R)-2,2,3,4,4,6,6,7,8-nonadeuterio-3,7-dihydroxy-10,13-dimethyl-5,9,11,12,14,15,16,17-octahydro-1H-cyclopenta[a]phenanthren-17-yl]pentanoic acid |
InChI |
InChI=1S/C24H40O4/c1-14(4-7-21(27)28)17-5-6-18-22-19(9-11-24(17,18)3)23(2)10-8-16(25)12-15(23)13-20(22)26/h14-20,22,25-26H,4-13H2,1-3H3,(H,27,28)/t14-,15+,16-,17-,18?,19?,20-,22?,23+,24-/m1/s1/i8D2,12D2,13D2,16D,20D,22D |
InChI Key |
RUDATBOHQWOJDD-NPFMQFSDSA-N |
Isomeric SMILES |
[2H][C@]1(C(C[C@]2([C@@H](C1([2H])[2H])C([C@@](C3(C2CC[C@]4(C3CC[C@@H]4[C@H](C)CCC(=O)O)C)[2H])([2H])O)([2H])[2H])C)([2H])[2H])O |
Canonical SMILES |
CC(CCC(=O)O)C1CCC2C1(CCC3C2C(CC4C3(CCC(C4)O)C)O)C |
Origin of Product |
United States |
Disclaimer and Information on In-Vitro Research Products
Please be aware that all articles and product information presented on BenchChem are intended solely for informational purposes. The products available for purchase on BenchChem are specifically designed for in-vitro studies, which are conducted outside of living organisms. In-vitro studies, derived from the Latin term "in glass," involve experiments performed in controlled laboratory settings using cells or tissues. It is important to note that these products are not categorized as medicines or drugs, and they have not received approval from the FDA for the prevention, treatment, or cure of any medical condition, ailment, or disease. We must emphasize that any form of bodily introduction of these products into humans or animals is strictly prohibited by law. It is essential to adhere to these guidelines to ensure compliance with legal and ethical standards in research and experimentation.