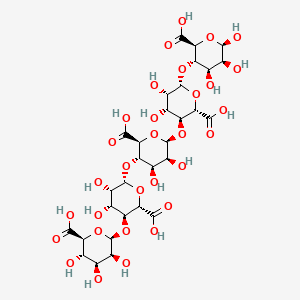
D-Pentamannuronic acid
- Click on QUICK INQUIRY to receive a quote from our team of experts.
- With the quality product at a COMPETITIVE price, you can focus more on your research.
Overview
Description
D-Pentamannuronic acid (C₃₀H₃₇O₃₁Na₅, MW: 1008.54 g/mol) is a sodium salt of an alginate oligosaccharide composed of five β-1,4-linked D-mannuronic acid residues . It is naturally derived from marine brown algae (e.g., Laminaria spp.) and certain Gram-negative bacteria (e.g., Pseudomonas aeruginosa) . This compound is widely utilized in biomedical research, particularly for studying neuroinflammatory conditions such as pain modulation and vascular dementia due to its immunomodulatory properties . Its commercial availability (e.g., CAS: 183668-50-0) is standardized at ≥95% purity, with storage recommendations at -20°C to ensure stability .
Preparation Methods
Enzymatic Hydrolysis of Alginate Polysaccharides
The most widely documented method involves enzymatic depolymerization of sodium alginate or alginic acid using alginate lyases. This approach yields oligomeric fragments, including pentamannuronic acid, with defined chain lengths.
Key Enzymes and Reaction Conditions
Mechanistic Insights
- β-Elimination : Alginate lyases cleave the glycosidic bonds between uronic acid residues, forming 4,5-unsaturated intermediates (Δ units) as non-reducing ends.
- Substrate Specificity : Enzymes like AlyRm3 exhibit bifunctional activity, cleaving both poly-M and poly-G blocks, with a preference for M-GM bonds in heteropolymeric regions.
Recombinant Alginate Lyase Engineering
To enhance enzymatic efficiency, researchers employ recombinant expression of truncated lyases. For example:
Case Study: Algt1 from Microbulbifer thermotolerans
Optimized Reaction Setup
- Substrate Preparation : 20% alginate solution in 20 mM Na₂HPO₄-NaH₂PO₄ buffer (pH 7.0).
- Enzyme Dosage : 10 U of Algt1 per mL.
- Incubation : 12 hours at 40°C.
- Product Analysis : TLC (thin-layer chromatography) and ESI-MS confirmed pentamannuronic acid as a primary product.
Chromatographic Purification
Post-hydrolysis purification is critical to isolate this compound.
Anion-Exchange Chromatography (AEX)
Workflow
- Crude Hydrolysate : Adjust pH to 2.85 to precipitate salts.
- Centrifugation : Remove insoluble residues.
- Ethanol Precipitation : Recover mannuronates from supernatant.
- AEX Chromatography : Elute with NaCl gradient to resolve oligomers by charge density.
Alternative Methods: Microbial Fermentation
While enzymatic methods dominate, microbial fermentation can produce alginate oligomers. For example:
Pseudomonas aeruginosa
- Mechanism : Exopolysaccharide degradation via endogenous lyases.
- Limitations : Low yield, complex downstream processing.
Quality Control and Characterization
Industrial-Scale Production Challenges
Chemical Reactions Analysis
Esterification Reactions
The carboxylic acid groups in D-pentamannuronic acid undergo esterification with alcohols under acidic or enzymatic conditions. This reaction modifies the compound's solubility and functional properties.
Reaction Mechanism :
D-Pentamannuronic acid+ROHH+or enzymeD-Pentamannuronic acid ester+H2O
-
Catalysts : Acidic conditions (e.g., HCl) or lipases.
-
Applications : Used to synthesize biodegradable polymers and drug delivery systems.
Acid-Catalyzed Hydrolysis
The glycosidic bonds between mannuronic acid units are cleaved under acidic conditions, yielding shorter oligomers or monomers.
Reaction Conditions :
Products :
-
Monomeric D-mannuronic acid and smaller oligomers (di-, tri-).
Enzymatic Hydrolysis
Alginate lyases selectively cleave glycosidic bonds via a β-elimination mechanism, producing unsaturated oligosaccharides.
Key Data from Pseudomonas sp. Lyase (PsAly) :
Parameter | Value | Source |
---|---|---|
Optimal Temperature | 65°C | |
Optimal pH | 7.0–7.5 | |
Metal Ion Activation | Mg²⁺, Mn²⁺, Co²⁺ enhance activity | |
Primary Products | Unsaturated tri-, tetra-, and pentamannuronates |
Mechanism :
PolymannuronatePsAlyΔ4,5-unsaturated oligomers
Note: The enzyme’s β-helix structure creates a substrate-binding cleft critical for catalysis .
Non-Enzymatic Glycation (Maillard Reaction)
This compound participates in glycation with amino groups, forming advanced glycation end products (AGEs).
Stages :
-
Initial Condensation :
D-Pentamannuronic acid+NH2→Schiff base→Amadori product -
Advanced Glycoxidation :
Reactive intermediates (e.g., methylglyoxal) crosslink proteins, forming irreversible AGEs .
Implications : Linked to oxidative stress in chronic diseases due to redox-active metal chelation .
Decarboxylation Pathways
Under thermal or enzymatic conditions, decarboxylation of mannuronic acid units may occur, though direct evidence for this compound is limited.
Theoretical Insights :
-
Quinone methide intermediates form via protonation of double bonds, followed by C–C bond cleavage .
-
Proposed active site residues (e.g., histidine) act as proton donors in analogous decarboxylases .
Oxidative Reactions
This compound’s reducing end undergoes oxidation, particularly in alkaline conditions, forming aldonic acids.
Example :
D-Pentamannuronic acid+O2pH > 9D-Mannonolactone+H2O2
Interaction with Divalent Cations
This compound forms gels via ionic crosslinking with Ca²⁺, Mg²⁺, or other divalent cations.
Gelation Mechanism :
2D-Pentamannuronic acid+Ca2+→“Egg-box” structure
Scientific Research Applications
D-Pentamannuronic acid has a wide range of scientific research applications. It is used in the study of pain and vascular dementia due to its biological activity . In chemistry, it serves as a model compound for studying alginate oligomers . In biology and medicine, it is used to investigate the mechanisms of action of alginate-derived compounds and their potential therapeutic applications . Additionally, it has industrial applications in the production of biocompatible materials and as a component in various biochemical assays .
Mechanism of Action
The mechanism of action of D-Pentamannuronic acid involves its interaction with specific molecular targets and pathways . It is believed to exert its effects by modulating the activity of certain enzymes and receptors involved in pain and vascular dementia . The exact molecular targets and pathways are still under investigation, but preliminary studies suggest that it may influence cytokine production and other cellular processes .
Comparison with Similar Compounds
Comparison with Structurally Similar Compounds
D-Pentamannuronic acid belongs to a family of D-mannuronic acid oligosaccharides that vary in chain length and sodium ion stoichiometry. Below is a detailed comparison with its homologs:
Structural and Physicochemical Properties
Property | D-Tetramannuronic Acid | This compound | D-Hexamannuronic Acid | D-Heptamannuronic Acid |
---|---|---|---|---|
Chain Length (units) | 4 | 5 | 6 | 7 |
Molecular Formula | C₂₄H₂₉O₂₆Na₄ | C₃₀H₃₇O₃₁Na₅ | C₃₆H₄₅O₃₆Na₆ | C₄₂H₅₃O₄₁Na₇ |
Molecular Weight (g/mol) | ~806.4 | 1008.54 | ~1210.6 | ~1412.7 |
Purity (%) | ≥98 | ≥95–97 | ≥95–96 | ≥95 |
Price (10 mg, USD) | 5720 | 1560 | 2179 | 2318 |
Primary Sources | Brown algae, bacteria | Brown algae, bacteria | Brown algae, bacteria | Brown algae, bacteria |
Key Observations :
- Chain Length vs. Molecular Weight : Each additional mannuronic acid unit increases molecular weight by ~200–202 g/mol, correlating with incremental sodium ions (e.g., tetramer: 4 Na⁺; pentamer: 5 Na⁺) .
- Purity Trends : Shorter oligomers (e.g., tetramer) exhibit higher purity (≥98%) compared to longer chains (e.g., heptamer: ≥95%), likely due to synthetic challenges in isolating longer oligosaccharides .
- Cost Implications : Despite its moderate purity, this compound is more cost-effective (1560 USD/10mg) than shorter or longer homologs, reflecting optimized production protocols .
Enzymatic Degradation
This compound and L-pentaguluronic acid (a guluronic acid pentamer) are both substrates for the recombinant alginate lyase AlgT1, which cleaves them into mono-, di-, tri-, and tetrasaccharides. However, this compound exhibits faster degradation kinetics compared to its guluronic counterpart, likely due to differential binding affinities in the enzyme’s active site .
Bioactivity in Disease Models
- Shared Applications: All D-mannuronic acid oligomers (tetra- to nonamers) are used to study neuroinflammatory pathways in vascular dementia and chronic pain .
- Chain-Length-Dependent Effects :
Commercial and Research Utility
Parameter | This compound | D-Guluronic Acid Oligomers | N-Acetylated Chitin Oligosaccharides |
---|---|---|---|
Source | Brown algae, bacteria | Brown algae | Crustacean shells, fungi |
Key Applications | Neuroinflammation, pain | Biomaterials, drug delivery | Immune modulation, plant defense |
Enzymatic Susceptibility | High (AlgT1) | Moderate (AlgT1) | Low (Chitinases) |
Cost (10 mg) | 1560 USD | Not reported | 3864–14874 USD |
Notable Contrasts:
- D-Guluronic acid oligomers are primarily used in biomaterial synthesis (e.g., hydrogels), whereas D-mannuronic acid derivatives focus on therapeutic research .
Biological Activity
D-Pentamannuronic acid, a polysaccharide derived from alginate, has garnered attention for its diverse biological activities. This article explores its biochemical properties, biological effects, and potential applications in various fields, including medicine and agriculture.
Structure and Properties
This compound is a polymer consisting of β-D-mannuronic acid units linked by β-(1,4) glycosidic bonds. It is one of the primary components of alginate, which is extracted from brown seaweeds. The structural configuration of this compound allows it to interact with various biological systems, influencing its activity.
Biological Activities
The biological activities of this compound can be categorized into several key areas:
1. Antioxidant Activity
- This compound exhibits significant antioxidant properties. Studies indicate that oligomannuronic acids derived from alginates can scavenge free radicals, thereby reducing oxidative stress in cells. This activity is crucial for protecting cells from damage caused by reactive oxygen species (ROS) .
2. Anti-inflammatory Effects
- Research has demonstrated that this compound can modulate inflammatory responses. It activates signaling pathways such as MAPK and NF-κB, leading to the release of anti-inflammatory cytokines. This property is particularly relevant in the context of neuroinflammation and conditions like Alzheimer's disease .
3. Immune Regulation
- This compound plays a role in immune modulation. It enhances the activity of immune cells, such as macrophages and microglia, improving their ability to respond to pathogens. The polysaccharide has shown promise in enhancing phagocytosis and cytokine production .
4. Antitumor Activity
- The compound has been investigated for its potential antitumor effects. Oligomannuronic acids have been reported to inhibit tumor growth and induce apoptosis in cancer cells. This activity is attributed to their ability to modulate cell signaling pathways involved in cell proliferation and survival .
Case Studies
Case Study 1: Neuroprotective Effects
A study evaluated the neuroprotective effects of a this compound derivative (MOS-Sia) on BV-2 microglial cells exposed to Aβ42 oligomers. The results indicated that MOS-Sia significantly reduced Aβ42-induced inflammation by inhibiting the release of pro-inflammatory cytokines (IL-1β, IL-6, TNF-α). Furthermore, lower concentrations of MOS-Sia enhanced cell viability and reduced apoptosis in BV-2 cells .
Case Study 2: Antioxidative Properties
In another investigation, the antioxidative capacity of this compound was assessed using rat brain tissues subjected to oxidative stress. The results demonstrated that treatment with oligomannuronic acids increased superoxide dismutase (SOD) levels, thereby protecting neurons from oxidative damage and improving cognitive functions .
The mechanisms underlying the biological activities of this compound are complex and involve multiple pathways:
- Antioxidant Mechanism: this compound's ability to donate electrons helps neutralize free radicals, thus preventing cellular damage.
- Inflammatory Modulation: It influences the expression of various genes involved in inflammation through activation of transcription factors like NF-κB.
- Immune Enhancement: By promoting macrophage activation and increasing cytokine production, it enhances the innate immune response.
Applications
Given its diverse biological activities, this compound has potential applications in:
- Pharmaceuticals: As a therapeutic agent for neurodegenerative diseases due to its neuroprotective and anti-inflammatory properties.
- Food Industry: As a functional ingredient with antioxidant properties that can enhance food preservation.
- Agriculture: As a biostimulant to promote plant growth and enhance resistance against pathogens.
Q & A
Basic Research Questions
Q. What established methods are recommended for confirming the structural integrity of D-Pentamannuronic acid in synthesized samples?
To validate the structure of this compound, researchers should employ a combination of nuclear magnetic resonance (NMR) spectroscopy and mass spectrometry (MS). NMR (e.g., ¹H, ¹³C, and 2D experiments like COSY and HSQC) can confirm the saccharide sequence and anomeric configurations, while MS (e.g., MALDI-TOF or ESI-MS) verifies molecular weight and purity. Chromatographic techniques like HPLC with refractive index or UV detection can assess homogeneity. Ensure protocols align with reproducibility standards by detailing solvent systems, column types, and calibration curves in the methodology .
Q. What in vitro assays are suitable for preliminary evaluation of this compound’s bioactivity?
Common assays include:
- Anti-inflammatory activity : Measure inhibition of pro-inflammatory cytokines (e.g., TNF-α, IL-6) in LPS-stimulated macrophages using ELISA.
- Antioxidant capacity : Use DPPH or ABTS radical scavenging assays, reporting IC₅₀ values.
- Neuronal protection : Assess viability in oxygen-glucose deprivation (OGD) models of vascular dementia via MTT assays. Include positive controls (e.g., ascorbic acid for antioxidants) and statistical validation (e.g., ANOVA with post-hoc tests) to ensure robustness .
Q. How should researchers design experiments to assess the stability of this compound under physiological conditions?
Simulate physiological environments by incubating the compound in buffers (e.g., PBS at pH 7.4) and biological fluids (e.g., serum) at 37°C. Monitor degradation over time using HPLC or capillary electrophoresis. For temperature-sensitive studies, employ accelerated stability testing (e.g., 40°C/75% RH). Report degradation kinetics (half-life, activation energy) and identify breakdown products via LC-MS .
Advanced Research Questions
Q. How can researchers reconcile discrepancies between in vitro and in vivo data on this compound’s pharmacological effects?
Conduct systematic pharmacokinetic/pharmacodynamic (PK/PD) studies to evaluate bioavailability, tissue distribution, and metabolite formation. Use techniques like microdialysis for real-time monitoring of unbound drug concentrations in target tissues (e.g., brain for vascular dementia). Validate in vitro-in vivo correlations (IVIVC) using compartmental modeling. Address interspecies differences by comparing rodent and human cell-based assays .
Q. What strategies optimize the resolution of this compound from complex biological matrices in HPLC analysis?
- Column selection : Use hydrophilic interaction liquid chromatography (HILIC) or ion-pair chromatography with tetrabutylammonium salts to enhance retention of polar acidic oligosaccharides.
- Sample preparation : Deproteinize matrices via ultrafiltration or solid-phase extraction (SPE) with activated charcoal.
- Detection : Couple HPLC with charged aerosol detection (CAD) or evaporative light scattering (ELS) for improved sensitivity. Validate recovery rates and limit of quantification (LOQ) using spiked samples .
Q. What molecular mechanisms underlie this compound’s reported neuroprotective effects?
Employ transcriptomics (RNA-seq) and proteomics (LC-MS/MS) to identify differentially expressed genes/proteins in treated neuronal cells. Validate targets (e.g., BDNF, Nrf2) via siRNA knockdown or CRISPR-Cas8. Use surface plasmon resonance (SPR) or isothermal titration calorimetry (ITC) to confirm direct binding to receptors like TLR4 or CD19. Correlate mechanistic findings with functional outcomes (e.g., reduced oxidative stress markers) .
Q. How can researchers address reproducibility challenges in synthesizing this compound with consistent chain length and sulfation patterns?
Standardize enzymatic depolymerization of alginate using lyases (e.g., AlyA1) with controlled reaction times and pH. Implement size-exclusion chromatography (SEC) or dialysis for precise molecular weight cutoff. Use ¹H NMR integration of anomeric protons to quantify polymerization degree. For sulfated derivatives, optimize reaction conditions (e.g., SO₃-pyridine ratio) and characterize via elemental analysis or FTIR .
Q. Data Analysis and Reporting
Q. What statistical approaches are critical for analyzing dose-response relationships in this compound studies?
Apply nonlinear regression models (e.g., four-parameter logistic curve) to calculate EC₅₀/IC₅₀ values. Use Bayesian hierarchical models for multi-experiment meta-analysis. Report confidence intervals and effect sizes (e.g., Cohen’s d) to quantify biological significance. For omics data, employ false discovery rate (FDR) correction and pathway enrichment tools (e.g., DAVID, KEGG) .
Q. How should researchers handle contradictory data on this compound’s immunomodulatory effects across different cell lines?
Perform sensitivity analysis to identify variables (e.g., cell passage number, serum batch). Use multi-omics integration (e.g., transcriptomics + metabolomics) to uncover context-dependent mechanisms. Replicate findings in primary cells or 3D organoid models. Transparently report conflicting data in discussion sections, emphasizing limitations and proposing follow-up experiments .
Properties
Molecular Formula |
C30H42O31 |
---|---|
Molecular Weight |
898.6 g/mol |
IUPAC Name |
(2S,3S,4S,5S,6R)-6-[(2S,3S,4R,5S,6R)-2-carboxy-6-[(2S,3S,4R,5S,6R)-2-carboxy-6-[(2S,3S,4R,5S,6R)-2-carboxy-6-[(2S,3S,4R,5S,6R)-2-carboxy-4,5,6-trihydroxyoxan-3-yl]oxy-4,5-dihydroxyoxan-3-yl]oxy-4,5-dihydroxyoxan-3-yl]oxy-4,5-dihydroxyoxan-3-yl]oxy-3,4,5-trihydroxyoxane-2-carboxylic acid |
InChI |
InChI=1S/C30H42O31/c31-1-2(32)16(21(42)43)58-27(8(1)38)55-13-4(34)10(40)29(60-18(13)23(46)47)57-15-6(36)11(41)30(61-20(15)25(50)51)56-14-5(35)9(39)28(59-19(14)24(48)49)54-12-3(33)7(37)26(52)53-17(12)22(44)45/h1-20,26-41,52H,(H,42,43)(H,44,45)(H,46,47)(H,48,49)(H,50,51)/t1-,2-,3+,4+,5+,6+,7-,8-,9-,10-,11-,12-,13-,14-,15-,16-,17-,18-,19-,20-,26+,27+,28+,29+,30+/m0/s1 |
InChI Key |
XTVQVZDWFHITEQ-YLWRECDYSA-N |
Isomeric SMILES |
[C@@H]1([C@@H]([C@H](O[C@H]([C@H]1O)O[C@H]2[C@@H]([C@@H]([C@@H](O[C@@H]2C(=O)O)O[C@H]3[C@@H]([C@@H]([C@@H](O[C@@H]3C(=O)O)O[C@H]4[C@@H]([C@@H]([C@@H](O[C@@H]4C(=O)O)O[C@H]5[C@@H]([C@@H]([C@@H](O[C@@H]5C(=O)O)O)O)O)O)O)O)O)O)O)C(=O)O)O)O |
Canonical SMILES |
C1(C(C(OC(C1O)OC2C(C(C(OC2C(=O)O)OC3C(C(C(OC3C(=O)O)OC4C(C(C(OC4C(=O)O)OC5C(C(C(OC5C(=O)O)O)O)O)O)O)O)O)O)O)C(=O)O)O)O |
Origin of Product |
United States |
Disclaimer and Information on In-Vitro Research Products
Please be aware that all articles and product information presented on BenchChem are intended solely for informational purposes. The products available for purchase on BenchChem are specifically designed for in-vitro studies, which are conducted outside of living organisms. In-vitro studies, derived from the Latin term "in glass," involve experiments performed in controlled laboratory settings using cells or tissues. It is important to note that these products are not categorized as medicines or drugs, and they have not received approval from the FDA for the prevention, treatment, or cure of any medical condition, ailment, or disease. We must emphasize that any form of bodily introduction of these products into humans or animals is strictly prohibited by law. It is essential to adhere to these guidelines to ensure compliance with legal and ethical standards in research and experimentation.