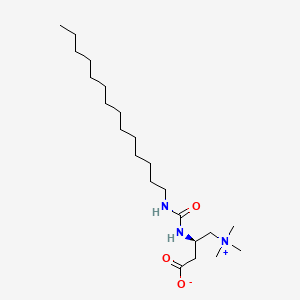
Teglicar
Overview
Description
Teglicar is a selective and reversible inhibitor of the liver isoform of carnitine palmitoyl-transferase 1 (L-CPT1). This enzyme plays a crucial role in the transport of long-chain fatty acids into the mitochondria for β-oxidation. This compound has shown potential antihyperglycemic properties and is being researched for its applications in diabetes and neurodegenerative diseases, including Huntington’s disease .
Preparation Methods
Synthetic Routes and Reaction Conditions
The synthesis of Teglicar involves multiple steps, starting with the preparation of key intermediates. The process typically includes:
Formation of the Core Structure: This involves the reaction of specific starting materials under controlled conditions to form the core structure of this compound.
Functional Group Modifications: Subsequent steps involve the introduction and modification of functional groups to achieve the desired chemical properties.
Purification: The final product is purified using techniques such as recrystallization or chromatography to ensure high purity.
Industrial Production Methods
Industrial production of this compound follows similar synthetic routes but is scaled up to meet demand. This involves optimizing reaction conditions to maximize yield and minimize impurities. Techniques such as continuous flow chemistry may be employed to enhance efficiency and scalability.
Chemical Reactions Analysis
Biochemical Mechanism of Action
Teglicar inhibits CPT1A, the rate-limiting enzyme for mitochondrial fatty acid β-oxidation, by competitively blocking the carnitine-binding site . This inhibition prevents the formation of acylcarnitines, effectively reducing fatty acid transport into mitochondria.
Key structural interactions :
-
Binds to CPT1A’s outer mitochondrial membrane domain
-
Reversible inhibition allows metabolic flexibility compared to irreversible inhibitors like etomoxir
Table 1: this compound’s Impact on Hepatic Glucose and Ketone Production
Concentration (μM) | Ketone Body Reduction (%) | Glucose Production Reduction (%) | Model System |
---|---|---|---|
1.0 | 16 | 8 | Rat hepatocytes |
3.0 | 32 | 17 | Rat hepatocytes |
10.0 | 72 | 50 | Rat hepatocytes |
17.5 | 84 | 62 | Canine CMT-U229 |
Notable findings :
-
Dose-dependent suppression of β-oxidation reduces acetyl-CoA availability for gluconeogenesis .
-
At 10 μM, this compound decreases glucose production by 50% in hepatocytes incubated with lactate/pyruvate substrates .
Table 2: Cytotoxic Effects in Canine Mammary Tumor Cells
Cell Line | This compound IC50 (μM) | Apoptosis Induction (vs. Control) | Caspase Activation Fold-Change |
---|---|---|---|
P114 | 5.5 | 92% at 30 μM | Caspase-9: 3.2x; Caspase-3: 4.1x |
CMT-U229 | 10.0 | 75% at 30 μM | Caspase-8: 2.8x; Caspase-3: 3.6x |
MDCK (normal) | >30 | <5% at 30 μM | No significant change |
Mechanistic insights :
-
Upregulates intrinsic (caspase-9) and extrinsic (caspase-8) apoptotic pathways .
-
Selective cytotoxicity toward cancer cells correlates with CPT1A overexpression .
In Vivo Metabolic Modulation
-
db/db mice : 45-day treatment (50 mg/kg BID) reduced fasting glucose by 50% and improved glucose tolerance (AUC ↓34%) .
-
Drosophila HD model : 50 μM this compound restored climbing ability by 13.5% (p < 0.01) and normalized carnitine cycle gene expression .
Stability and Reactivity Data
No direct synthetic or degradation pathway studies were identified in the reviewed literature. Current data focus on its pharmacologic interactions rather than intrinsic chemical reactivity.
Scientific Research Applications
Antidiabetic Applications
Mechanism of Action
Teglicar functions by selectively inhibiting liver carnitine palmitoyltransferase 1, leading to reduced gluconeogenesis and improved glucose homeostasis. This mechanism is crucial for managing hyperglycemia, especially in type 2 diabetes patients.
Research Findings
- In studies involving C57BL/6J and db/db mice, this compound administration significantly decreased postabsorptive serum glucose levels by up to 50% after 28 days of treatment. Additionally, it reduced serum fructosamine and daily water consumption, indicating improved long-term glycemic control .
- This compound's effect on glucose production was demonstrated in isolated hepatocytes, where it reduced both ketone bodies and glucose production in a concentration-dependent manner .
Table 1: Effects of this compound on Glycemic Control
Study Type | Dosage (mg/kg) | Duration (days) | Glucose Reduction (%) | Insulin Reduction (%) |
---|---|---|---|---|
C57BL/6J Mice | 50 (twice/day) | 45 | 50 | 60 |
db/db Mice | 50 (twice/day) | 45 | 50 | Not specified |
Healthy Rats | 80 | 30 | 20 (ns) | Not specified |
Cancer Therapeutics
Antitumor Activity
this compound has shown promise in the treatment of various cancers by inducing apoptosis in cancer cells. Its application extends to hematological malignancies and solid tumors.
Case Studies
- A study reported that combining this compound with venetoclax enhanced apoptosis in multiple myeloma cells, suggesting a synergistic effect that may improve treatment outcomes .
- In canine mammary cancer models, this compound treatment significantly inhibited cell viability, demonstrating its potential as a therapeutic agent against certain types of tumors .
Table 2: Antitumor Effects of this compound
Cancer Type | Dosage (µM) | Viability Reduction (%) | Apoptosis Induction (%) |
---|---|---|---|
Multiple Myeloma | Not specified | Significant | Yes |
Canine Mammary Cancer | 10-30 | Up to 92 | Early and late-stage |
Neuroprotective Effects
Emerging research indicates that this compound may have neuroprotective properties. In models of Huntington's disease using Drosophila, chronic treatment with this compound improved motor function compared to untreated controls . This suggests potential applications in neurodegenerative diseases.
Mechanism of Action
Teglicar exerts its effects by selectively inhibiting the liver isoform of carnitine palmitoyl-transferase 1 (L-CPT1). This inhibition reduces the transport of long-chain fatty acids into the mitochondria, thereby decreasing β-oxidation and ketogenesis. The reduction in fatty acid oxidation leads to lower glucose production and improved glucose homeostasis .
Comparison with Similar Compounds
Similar Compounds
Etomoxir: Another carnitine palmitoyl-transferase 1 inhibitor, but it is irreversible and has different pharmacokinetic properties.
Perhexiline: Inhibits carnitine palmitoyl-transferase 1 and 2, used primarily for angina treatment.
Meldonium: Inhibits carnitine biosynthesis, affecting fatty acid metabolism.
Uniqueness of Teglicar
This compound’s selectivity and reversibility make it unique among carnitine palmitoyl-transferase 1 inhibitors. Its oral bioavailability and potential antihyperglycemic properties further distinguish it from similar compounds .
Biological Activity
Teglicar, a selective reversible inhibitor of carnitine palmitoyltransferase 1 (CPT1), has garnered attention for its diverse biological activities, particularly in the realms of diabetes management and cancer therapy. This article synthesizes findings from various studies, highlighting its mechanisms of action, effects on glucose metabolism, and antitumor properties.
This compound functions primarily by inhibiting CPT1, an enzyme critical for fatty acid oxidation. By blocking this pathway, this compound reduces the availability of acetyl-CoA in the cytosol, subsequently decreasing gluconeogenesis (GNG) and promoting glucose oxidation. This mechanism is pivotal in conditions such as diabetes and certain cancers where metabolic dysregulation is prevalent.
In Vitro Studies
In isolated hepatocytes, this compound demonstrated a concentration-dependent reduction in both ketone body and glucose production. Specifically, at a concentration of 10 μmol/L, ketone body production was reduced by 72%, while cumulative glucose production decreased by 50% compared to untreated cells . This suggests that this compound effectively inhibits GNG, a key contributor to hyperglycemia in diabetic patients.
Animal Models
In studies involving diabetic animal models, such as db/db mice and SD rats , this compound significantly reduced endogenous glucose production (EGP) by up to 62% without affecting peripheral glucose utilization . Long-term administration (50 mg/kg twice daily for 45 days) led to a notable decrease in postabsorptive serum glucose levels by approximately 50%, alongside reductions in fructosamine levels, indicating improved glycemic control .
Table: Summary of this compound's Effects on Glucose Metabolism
Study Type | Model | Dosage | EGP Reduction | Serum Glucose Reduction | Other Effects |
---|---|---|---|---|---|
In Vitro | Isolated Hepatocytes | 10 μmol/L | 72% (ketone) | 50% (glucose) | Concentration-dependent |
Animal Study | db/db Mice | 50 mg/kg twice daily | 62% | 50% | Reduced fructosamine |
Long-term Treatment | SD Rats | 80 mg/kg | Not specified | ~20% | Increased triglycerides |
Antitumor Activity
This compound has also been investigated for its potential antitumor effects. Studies indicate that it can induce apoptosis in various cancer cell lines, including HeLa cervical cancer cells and canine mammary tumor cells. The mechanism involves endoplasmic reticulum stress pathways and activation of caspases, leading to programmed cell death .
Case Studies
- HeLa Cells : In vitro experiments showed that this compound reduced cell viability significantly through apoptosis induction via ER stress pathways .
- Canine Mammary Tumors : this compound treatment resulted in a marked decrease in cell viability and induced morphological changes consistent with apoptosis. The expression of pro-apoptotic genes such as caspase-3 was significantly upregulated following treatment .
Table: Antitumor Effects of this compound
Cancer Type | Cell Line | Dosage | Viability Reduction | Mechanism |
---|---|---|---|---|
Cervical Cancer | HeLa | Not specified | Significant | Apoptosis via ER stress |
Canine Mammary Tumors | CMT-U229 | 17.5 μM | Significant | Caspase activation |
Q & A
Basic Research Questions
Q. How can researchers design reproducible experimental protocols for studying Teglicar’s pharmacokinetic properties?
- Methodological Answer : Begin by defining measurable endpoints (e.g., bioavailability, half-life) and selecting appropriate in vitro (e.g., hepatic microsomes) and in vivo (rodent models) systems. Use standardized buffers and solvents to minimize variability. For reproducibility, follow guidelines from journals like the Beilstein Journal of Organic Chemistry, which emphasize detailed experimental descriptions, including solvent purity, temperature controls, and equipment calibration . Validate results using triplicate runs and include negative controls to account for metabolic interference.
Q. What are the best practices for conducting a systematic literature review on this compound’s mechanism of action?
- Methodological Answer : Use Google Scholar’s advanced search operators (e.g.,
"this compound" AND "mechanism of action"
) to filter peer-reviewed articles . Prioritize studies with robust methodologies, such as those employing crystallography, NMR, or molecular docking. Organize findings into a matrix comparing experimental conditions (e.g., pH, concentration) and outcomes. Critically evaluate contradictions by cross-referencing with databases like PubMed and citing primary sources to avoid reliance on review articles .
Q. How should researchers address variability in this compound’s solubility data across studies?
- Methodological Answer : Perform solubility assays under standardized conditions (e.g., USP phosphate buffer, 37°C) and document solvent purity, agitation methods, and equilibration time. Compare results with existing data using Bland-Altman plots to identify systematic biases. If contradictions persist, conduct meta-analyses to quantify heterogeneity and propose consensus protocols .
Advanced Research Questions
Q. How can contradictory findings about this compound’s off-target effects be resolved?
- Methodological Answer : Design a multi-omics approach:
Use transcriptomics (RNA-seq) to identify differentially expressed genes post-Teglicar exposure.
Validate findings with proteomics (LC-MS/MS) to confirm protein-level changes.
Apply cheminformatics tools (e.g., SwissTargetPrediction) to predict off-target interactions.
Cross-validate results using orthogonal assays (e.g., SPR for binding affinity) and publish raw data in repositories like Zenodo to enable independent verification .
Q. What statistical methods are appropriate for analyzing dose-response relationships in this compound toxicity studies?
- Methodological Answer : Fit data to nonlinear regression models (e.g., Hill equation) using software like GraphPad Prism. Report IC₅₀ values with 95% confidence intervals and assess goodness-of-fit via R² and residual plots. For non-monotonic responses, use Bayesian hierarchical models to account for heteroscedasticity .
Q. How can researchers optimize this compound’s synthesis protocol to improve yield and purity?
- Methodological Answer : Apply Design of Experiments (DoE) principles:
- Factors : Catalyst loading, reaction temperature, solvent polarity.
- Responses : Yield (%), HPLC purity (area %).
Use a Central Composite Design to identify optimal conditions and validate with three independent batches. Characterize intermediates via LC-MS and XRD to trace impurity sources .
Q. Data Contradiction Analysis
Q. Why do studies report conflicting results about this compound’s stability under physiological conditions?
- Methodological Answer : Stability discrepancies often arise from:
- Matrix effects : Plasma vs. buffer systems alter degradation kinetics.
- Analytical methods : HPLC-UV vs. LC-MS/MS vary in sensitivity to degradation products.
Resolve by replicating experiments under cited conditions and using forced degradation studies (e.g., exposure to light, heat) to identify critical stability factors .
Q. Methodological Tables
Characterization Technique | Key Parameters | Relevance to this compound |
---|---|---|
LC-MS/MS | Mass accuracy, fragmentation | Quantifies metabolites and degradation products |
Isothermal Titration Calorimetry | ΔH, Kd | Measures binding affinity to target proteins |
XRD | Crystal lattice parameters | Confirms polymorphic forms and purity |
Properties
IUPAC Name |
(3R)-3-(tetradecylcarbamoylamino)-4-(trimethylazaniumyl)butanoate | |
---|---|---|
Source | PubChem | |
URL | https://pubchem.ncbi.nlm.nih.gov | |
Description | Data deposited in or computed by PubChem | |
InChI |
InChI=1S/C22H45N3O3/c1-5-6-7-8-9-10-11-12-13-14-15-16-17-23-22(28)24-20(18-21(26)27)19-25(2,3)4/h20H,5-19H2,1-4H3,(H2-,23,24,26,27,28)/t20-/m1/s1 | |
Source | PubChem | |
URL | https://pubchem.ncbi.nlm.nih.gov | |
Description | Data deposited in or computed by PubChem | |
InChI Key |
BMZYTDRMCBZVNH-HXUWFJFHSA-N | |
Source | PubChem | |
URL | https://pubchem.ncbi.nlm.nih.gov | |
Description | Data deposited in or computed by PubChem | |
Canonical SMILES |
CCCCCCCCCCCCCCNC(=O)NC(CC(=O)[O-])C[N+](C)(C)C | |
Source | PubChem | |
URL | https://pubchem.ncbi.nlm.nih.gov | |
Description | Data deposited in or computed by PubChem | |
Isomeric SMILES |
CCCCCCCCCCCCCCNC(=O)N[C@H](CC(=O)[O-])C[N+](C)(C)C | |
Source | PubChem | |
URL | https://pubchem.ncbi.nlm.nih.gov | |
Description | Data deposited in or computed by PubChem | |
Molecular Formula |
C22H45N3O3 | |
Source | PubChem | |
URL | https://pubchem.ncbi.nlm.nih.gov | |
Description | Data deposited in or computed by PubChem | |
Molecular Weight |
399.6 g/mol | |
Source | PubChem | |
URL | https://pubchem.ncbi.nlm.nih.gov | |
Description | Data deposited in or computed by PubChem | |
CAS No. |
250694-07-6 | |
Record name | Teglicar [INN] | |
Source | ChemIDplus | |
URL | https://pubchem.ncbi.nlm.nih.gov/substance/?source=chemidplus&sourceid=0250694076 | |
Description | ChemIDplus is a free, web search system that provides access to the structure and nomenclature authority files used for the identification of chemical substances cited in National Library of Medicine (NLM) databases, including the TOXNET system. | |
Record name | TEGLICAR | |
Source | FDA Global Substance Registration System (GSRS) | |
URL | https://gsrs.ncats.nih.gov/ginas/app/beta/substances/8V3VQ6HMII | |
Description | The FDA Global Substance Registration System (GSRS) enables the efficient and accurate exchange of information on what substances are in regulated products. Instead of relying on names, which vary across regulatory domains, countries, and regions, the GSRS knowledge base makes it possible for substances to be defined by standardized, scientific descriptions. | |
Explanation | Unless otherwise noted, the contents of the FDA website (www.fda.gov), both text and graphics, are not copyrighted. They are in the public domain and may be republished, reprinted and otherwise used freely by anyone without the need to obtain permission from FDA. Credit to the U.S. Food and Drug Administration as the source is appreciated but not required. | |
Retrosynthesis Analysis
AI-Powered Synthesis Planning: Our tool employs the Template_relevance Pistachio, Template_relevance Bkms_metabolic, Template_relevance Pistachio_ringbreaker, Template_relevance Reaxys, Template_relevance Reaxys_biocatalysis model, leveraging a vast database of chemical reactions to predict feasible synthetic routes.
One-Step Synthesis Focus: Specifically designed for one-step synthesis, it provides concise and direct routes for your target compounds, streamlining the synthesis process.
Accurate Predictions: Utilizing the extensive PISTACHIO, BKMS_METABOLIC, PISTACHIO_RINGBREAKER, REAXYS, REAXYS_BIOCATALYSIS database, our tool offers high-accuracy predictions, reflecting the latest in chemical research and data.
Strategy Settings
Precursor scoring | Relevance Heuristic |
---|---|
Min. plausibility | 0.01 |
Model | Template_relevance |
Template Set | Pistachio/Bkms_metabolic/Pistachio_ringbreaker/Reaxys/Reaxys_biocatalysis |
Top-N result to add to graph | 6 |
Feasible Synthetic Routes
Disclaimer and Information on In-Vitro Research Products
Please be aware that all articles and product information presented on BenchChem are intended solely for informational purposes. The products available for purchase on BenchChem are specifically designed for in-vitro studies, which are conducted outside of living organisms. In-vitro studies, derived from the Latin term "in glass," involve experiments performed in controlled laboratory settings using cells or tissues. It is important to note that these products are not categorized as medicines or drugs, and they have not received approval from the FDA for the prevention, treatment, or cure of any medical condition, ailment, or disease. We must emphasize that any form of bodily introduction of these products into humans or animals is strictly prohibited by law. It is essential to adhere to these guidelines to ensure compliance with legal and ethical standards in research and experimentation.