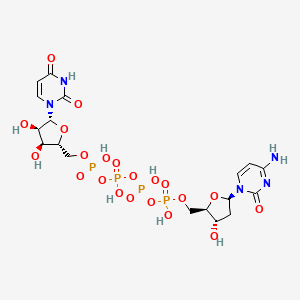
Denufosol
Overview
Description
Denufosol tetrasodium is a P2Y2 receptor agonist developed to address the ion transport defect in cystic fibrosis (CF), a genetic disorder characterized by dysfunctional CFTR chloride channels. This compound enhances chloride secretion via calcium-activated chloride channels (CaCCs) and inhibits epithelial sodium channel (ENaC) activity, thereby restoring airway surface liquid (ASL) depth and mucociliary clearance (MCC) . Preclinical studies demonstrated its ability to improve MCC in CF models, leading to clinical trials targeting early-stage CF patients with preserved lung function (FEV1 ≥75%) .
Preparation Methods
Chemical Synthesis of Denufosol
This compound’s structure comprises uridine and cytidine moieties linked by a tetraphosphate bridge. Its synthesis requires precise control over phosphorylation and coupling reactions to ensure regioselectivity and purity.
Phosphoromorpholidate Intermediate Method
Early synthetic approaches for dinucleoside polyphosphates, including this compound, utilized phosphoromorpholidate intermediates. As outlined in dinucleotide synthesis reviews , this method involves activating nucleoside monophosphates (NMPs) with reagents like -dicyclohexylcarbodiimide (DCC) in the presence of morpholine. For this compound, uridine monophosphate (UMP) and cytidine monophosphate (CMP) are converted into their morpholidate derivatives, which subsequently react with pyrophosphate to form the tetraphosphate bridge.
Key Reaction Conditions
-
Activation: UMP or CMP is treated with DCC and morpholine in anhydrous dimethylformamide (DMF) at 4°C for 12–24 hours.
-
Coupling: The activated morpholidate reacts with pyrophosphate () in the presence of magnesium chloride () to facilitate nucleophilic attack.
-
Yield: Early protocols reported yields of 10–40%, necessitating iterative optimization for industrial-scale production .
Imidazolide Activation Strategy
A more efficient method employs -carbonyldiimidazole (CDI) to activate phosphate groups. This approach, validated in boranophosphate isostere studies , involves:
-
Activation: The tri--butylammonium salt of UMP or CMP reacts with CDI in DMF to form a phosphorimidazolide intermediate.
-
Coupling: The intermediate reacts with a second nucleotide (e.g., cytidine triphosphate) under anhydrous conditions, with as a catalyst.
-
Purification: Reverse-phase HPLC isolates this compound from unreacted substrates and byproducts .
Advantages
-
Higher regioselectivity due to CDI’s specificity for primary phosphate groups.
-
Reduced reaction times (3–6 hours vs. 24 hours for morpholidate methods).
Limitations
-
Sensitivity to moisture, requiring stringent anhydrous conditions.
-
Byproduct formation (e.g., imidazole derivatives) necessitates additional purification steps .
Boranophosphate Isostere Modifications
To enhance metabolic stability, boranophosphate analogues of this compound were explored . These syntheses involve:
-
Phosphitylation: 2′,3′-Protected nucleosides are treated with salicylchlorophosphite to form cyclic intermediates.
-
Boration: A borane–dimethyl sulfide complex introduces boranophosphate groups at the - or -positions of the phosphate backbone.
-
Deprotection: Acidic hydrolysis (pH 2.3) removes protecting groups, yielding the final product.
While boranophosphate derivatives showed improved enzymatic resistance, their clinical relevance remains underexplored compared to native this compound .
Formulation and Lyophilization
This compound’s instability in aqueous solutions necessitates lyophilization for long-term storage. Patent data delineate critical formulation parameters:
Nebulization-Compatible Solutions
For inhalation, this compound is reconstituted in sterile saline at concentrations of 18–65 mg/mL. Key considerations include:
-
Osmolarity: Adjusted to 250–350 mOsm/kg to minimize airway irritation.
-
pH: Maintained at 6.8–7.4 using sodium phosphate buffer.
Lyophilization Process
A patented lyophilization protocol involves:
-
Freezing: The this compound solution is rapidly cooled to −50°C to form amorphous ice.
-
Primary Drying: Sublimation under vacuum (0.1 mBar) at −30°C removes 95% of water.
-
Secondary Drying: Residual moisture (<1%) is eliminated by heating to 25°C under reduced pressure.
Critical Quality Attributes
-
Residual Solvents: Acetone and DMF levels <0.5% (ICH guidelines).
-
Reconstitution Time: <2 minutes in 3 mL sterile water.
Analytical Characterization
Purity Assessment
This compound batches are analyzed via:
-
HPLC: C18 column (4.6 × 250 mm), mobile phase of 50 mM ammonium acetate (pH 5.0)/acetonitrile (95:5), UV detection at 260 nm.
-
Mass Spectrometry: ESI-MS confirms the molecular ion at 773.323 [M−H] .
Stability Studies
Accelerated stability testing (40°C/75% RH for 6 months) revealed:
-
Degradation Products: <2% uridine monophosphate and cytidine triphosphate.
Industrial-Scale Challenges
Cost of Raw Materials
The tri--butylammonium salts of nucleotides, used in CDI activation, contribute to 60–70% of production costs. Alternatives like tetrabutylammonium hydroxide are being explored .
Regulatory Considerations
Chemical Reactions Analysis
Table 1: Key structural properties
The molecule consists of uridine and 2'-deoxycytidine linked via a tetraphosphate chain, forming a dinucleoside polyphosphate scaffold . Its stability in aqueous solutions is pH-dependent, requiring sodium counterions to maintain solubility .
Synthetic Reactions
This compound is synthesized through phosphoramidite chemistry or enzymatic ligation , with key steps including:
-
Phosphate coupling : Sequential activation of nucleoside hydroxyl groups using reagents like 1H-tetrazole and 2-chloro-5,5-dimethyl-1,3,2-dioxaphosphorinane-2-oxide to form the tetraphosphate bridge .
-
Deprotection : Acidic or basic hydrolysis to remove protective groups (e.g., cyanoethyl or tert-butyl).
-
Salt formation : Neutralization with sodium hydroxide to yield the tetrasodium salt .
Table 2: Synthetic challenges and solutions
Stability and Degradation
This compound undergoes hydrolysis under acidic or alkaline conditions, cleaving the tetraphosphate chain into mononucleotides . Key stability data:
Table 3: Degradation pathways
Condition | Degradation Product | Half-Life | Source |
---|---|---|---|
pH < 5 | Uridine 5'-monophosphate + Deoxycytidine 5'-monophosphate | <24 hrs | |
pH > 8 | Cyclic phosphate intermediates | 48–72 hrs | |
High humidity | Partial hydrolysis | N/A |
Lyophilization or spray-drying with cryoprotectants (e.g., mannitol) is required for long-term storage .
Chemical Interactions
This compound’s reactivity is dominated by its phosphate backbone and nucleobases :
-
Metal chelation : The tetraphosphate chain binds divalent cations (e.g., Ca²⁺, Mg²⁺), potentially altering bioavailability .
-
Oxidation : The pyrimidine rings (uracil and cytosine) are susceptible to oxidative damage by reactive oxygen species .
No evidence of hepatic metabolism or cytochrome P450 interactions exists, as systemic absorption is minimal after inhalation .
Table 4: Key analytical methods
Method | Application | Source |
---|---|---|
Reverse-phase HPLC | Purity assessment (≥98%) | |
Mass spectrometry (ESI-MS) | Molecular weight confirmation | |
³¹P-NMR | Phosphate linkage verification |
Scientific Research Applications
Clinical Trials and Efficacy
Denufosol has undergone multiple clinical trials to evaluate its safety and efficacy in patients with cystic fibrosis. Notably:
- Phase 2 Trial : A randomized study demonstrated that this compound significantly improved lung function in patients with mild cystic fibrosis lung disease. The trial involved 89 patients who received nebulized this compound three times daily for 28 days, showing significant improvements in Forced Expiratory Volume (FEV1) compared to placebo (P = 0.006) .
- Phase 3 Trial (TIGER-1) : This pivotal trial included 352 patients with normal to mildly impaired lung function. Results indicated a statistically significant improvement in FEV1 at the 24-week endpoint, with a mean change of 0.048 L for this compound compared to 0.003 L for placebo (P = 0.047). The trial also highlighted this compound's safety profile, which was comparable to that of placebo .
- Long-term Outcomes : In the open-label extension of the TIGER-1 trial, patients continued to show progressive improvements in lung function, suggesting potential long-term benefits of this compound therapy .
Case Study Insights
- Patient Response Variability : In a subset analysis from the Phase 3 TIGER-1 trial, responses to this compound varied among patients based on baseline lung function metrics. Patients with higher baseline FEV1 showed more pronounced improvements, emphasizing the need for personalized treatment approaches .
- Adverse Events Profile : The most common adverse event reported was cough, occurring in approximately 47% of this compound patients versus 52% in placebo patients. Overall, this compound was well tolerated across studies, with no significant differences in adverse events when compared to placebo .
Data Summary Table
Study Phase | Patient Population | Duration | Primary Endpoint | Outcome |
---|---|---|---|---|
Phase 2 | 89 mild CF patients | 28 days | Change in FEV1 | Significant improvement (P = 0.006) |
Phase 3 (TIGER-1) | 352 CF patients | 24 weeks | Change in FEV1 | Significant improvement (P = 0.047) |
Open-label Extension | Same cohort | Additional weeks | Continued change in FEV1 | Progressive improvement observed |
Mechanism of Action
Denufosol exerts its effects by acting as an agonist at the P2Y2 subtype of purinergic receptors. This activation leads to the stimulation of an alternative chloride channel, which increases chloride and water secretion on the epithelial cell surface. This mechanism enhances mucociliary clearance by increasing ciliary beat frequency and mucin secretion. The molecular targets involved include the P2Y2 receptor and associated G proteins .
Comparison with Similar Compounds
Clinical Development :
- Phase 2 trials (2005–2007) showed significant FEV1 improvements (e.g., +0.048L vs. +0.003L for placebo over 28 days) .
- Phase 3 trials (TIGER-1 and TIGER-2) initially reported positive results but ultimately failed to demonstrate sustained efficacy in long-term studies (48 weeks), leading to discontinuation . Key limitations included its short half-life (17 minutes) and transient pharmacodynamic effects .
P2Y2 Receptor Agonists
UTP and ATP
- Mechanism: Endogenous P2Y2 agonists that activate CaCCs and inhibit ENaC.
- Limitations : Rapid extracellular degradation (minutes) limits therapeutic utility .
- Comparison : Denufosol was engineered for metabolic stability, with a longer duration of action (hours vs. minutes for UTP/ATP) .
Moli-1901 (Lantibio)
- Mechanism : Elevates intracellular calcium independently of P2Y2 receptors, indirectly activating CaCCs .
ENaC Inhibitors
Amiloride
- Mechanism : Directly blocks ENaC to reduce sodium hyperabsorption and retain ASL .
- Clinical Outcomes : Failed in Phase 3 trials due to transient binding and insufficient efficacy .
- Comparison : this compound’s dual action (ENaC inhibition + CaCC activation) provided a broader mechanism but faced similar challenges in sustaining effects .
Alternative Chloride Secretagogues
Lubiprostone
- Mechanism : Activates CLC-2 chloride channels, independent of CFTR or CaCCs .
- Status: Approved for constipation but explored off-label in CF. Limited efficacy in CF trials, possibly due to non-airway targets .
- Comparison : this compound’s airway-specific delivery (via nebulization) offered targeted action but lacked prolonged retention .
Clinical Trial Outcomes and Pharmacokinetics
Table 1: Efficacy and Pharmacokinetic Comparison
Key Findings:
- This compound’s Early Success : Phase 2 trials demonstrated acute FEV1 improvements (e.g., +0.15L in mild CF patients over 28 days ), attributed to transient CaCC activation .
- −3.04% for placebo) or exacerbation rates . Pharmacokinetic sub-studies revealed insufficient drug exposure due to rapid clearance .
Lessons from this compound’s Development
- Mechanistic Redundancy : Newer CFTR modulators (e.g., ivacaftor, lumacaftor) target the root cause of CF and have overshadowed this compound’s alternative chloride secretion approach .
Biological Activity
Denufosol tetrasodium is a selective P2Y2 receptor agonist primarily investigated for its potential in treating cystic fibrosis (CF) through enhancing mucosal hydration and mucociliary clearance in the lungs. This article reviews the biological activity of this compound, focusing on its pharmacodynamics, clinical efficacy, and safety profile based on diverse research studies.
This compound functions by stimulating the P2Y2 receptor, which leads to:
- Increased Chloride Secretion : Activates alternative chloride channels that bypass the defective cystic fibrosis transmembrane conductance regulator (CFTR) channel.
- Enhanced Mucosal Hydration : Promotes liquid secretion on the epithelial surface, thereby improving airway hydration.
- Ciliary Function Enhancement : Increases cilia beat frequency, facilitating better mucus clearance from the airways.
- Mucin Secretion Stimulation : Encourages the release of mucins, which are critical for maintaining airway surface liquid and trapping pathogens.
These mechanisms collectively contribute to improved lung function in patients with CF by restoring normal airway hydration and clearance processes .
Phase 2 Trials
A pivotal Phase 2 randomized controlled trial assessed the safety and efficacy of this compound in patients with mild CF. The study involved 89 patients who received either this compound (20, 40, or 60 mg) or placebo over 28 days. Key findings included:
- Lung Function Improvement : Patients receiving this compound showed statistically significant improvements in forced expiratory volume (FEV1), forced expiratory flow (FEF25%-75%), and forced vital capacity (FVC) compared to placebo (P = 0.006 for FEV1) .
- Adverse Events : The most common side effect was cough, reported by 52% of placebo and 47% of this compound patients. Overall, this compound was well tolerated with no significant dose-response trends in adverse events .
Phase 3 Trials
This compound was also evaluated in Phase 3 trials, although these studies faced challenges in demonstrating consistent efficacy across broader patient populations. Despite initial promising results, subsequent trials indicated a lack of significant improvement in lung function metrics compared to placebo controls, leading to the cessation of further development in 2011 .
Pharmacokinetics
This compound exhibits a favorable pharmacokinetic profile characterized by:
- Limited Systemic Absorption : Studies indicated that nebulized doses resulted in minimal systemic exposure while achieving high concentrations in the lungs.
- Inhalation Delivery : Approximately 7 mg of a 40 mg nebulizer load was deposited in the lungs during radiolabeled studies, highlighting efficient delivery to target tissues .
Case Studies and Observations
A review of multiple studies highlighted that this compound's unique action as a P2Y2 agonist differentiates it from other CF treatments. In vitro studies demonstrated that ATP and UTP could restore liquid transport in CF cultures, supporting this compound's mechanism of action. However, real-world applications showed variability in responses among different patient cohorts .
Summary Table of Clinical Findings
Study Type | Patient Population | Treatment Duration | Key Findings | Adverse Events |
---|---|---|---|---|
Phase 2 Trial | Mild CF Patients | 28 days | Significant improvement in FEV1 (P = 0.006) | Cough (47% this compound) |
Phase 3 Trial | Moderate to Severe CF | Variable | No significant improvement over placebo | Similar adverse events |
Q & A
Basic Research Questions
Q. What is the molecular mechanism by which Denufosol modulates chloride transport in cystic fibrosis (CF) airway epithelia?
this compound, a P2Y₂ receptor agonist, activates purinergic signaling by binding to apical P2Y₂ receptors on airway epithelial cells. This triggers phospholipase C-mediated hydrolysis of phosphatidylinositol 4,5-bisphosphate (PIP₂), generating inositol trisphosphate (IP₃) and increasing intracellular Ca²⁺. Elevated Ca²⁺ activates calcium-sensitive chloride channels (CaCCs), bypassing the defective CFTR channel in CF patients. Concurrently, PIP₂ depletion inhibits the epithelial sodium channel (ENaC), reducing Na⁺ absorption and improving airway surface liquid (ASL) hydration . In vitro models using CF bronchial epithelial cultures demonstrated increased chloride secretion and mucociliary clearance .
Q. What in vitro and preclinical models are most relevant for evaluating this compound’s efficacy?
Primary human bronchial epithelial (HBE) cell cultures from CF patients are the gold standard for assessing ion transport and ASL height. Electrophysiological measurements (e.g., Ussing chamber) quantify chloride secretion and sodium absorption. Preclinical CF mouse models, though lacking spontaneous lung disease, are used to study pharmacokinetics and mucociliary clearance via biomarkers like DTPA aerosol retention . Co-administration with hypertonic saline or DNase has been tested to evaluate synergistic effects on mucus viscosity .
Q. How were early-phase clinical trials designed to assess this compound’s safety and efficacy?
Phase I trials focused on safety and tolerability in healthy volunteers and CF patients, using spirometry (FEV₁) and adverse event monitoring. Phase II trials employed randomized, placebo-controlled designs with endpoints including FEV₁ change, mucociliary clearance rates (measured via radiolabeled tracer retention), and sputum biomarkers. The TIGER-1 trial (Phase III) stratified patients by baseline FEV₁ (>75% predicted) and used a 60 mg t.i.d. regimen via Pari LC Star nebulizer. Substudies analyzed pharmacokinetics (e.g., plasma half-life: ~17 minutes) and receptor desensitization .
Advanced Research Questions
Q. Why did this compound fail in Phase III trials despite promising Phase II results?
The TIGER-2 trial (Phase III) failed to replicate TIGER-1’s FEV₁ improvements due to several factors:
- Pharmacokinetic Limitations : The short half-life (17 minutes) led to transient receptor activation, insufficient for sustained CaCC stimulation .
- Endpoint Selection : FEV₁ improvement may not capture long-term decline mitigation. Post-hoc analyses suggested lung function stabilization rather than enhancement .
- Receptor Desensitization : Chronic P2Y₂ activation downregulated signaling pathways, reducing efficacy over time .
- Population Heterogeneity : Patients with advanced disease (FEV₁ <75%) showed minimal response, highlighting the need for early intervention .
Q. What methodological approaches can address this compound’s pharmacokinetic limitations?
- Nebulizer Optimization : Pari eFlow® or vibrating mesh nebulizers could enhance aerosol delivery efficiency compared to the Pari LC Star .
- Dosing Interval Adjustments : Shorter intervals (e.g., q.i.d.) or sustained-release formulations might counteract rapid clearance .
- Biomarker-Driven Dosing : Real-time ASL pH or viscosity monitoring could personalize dosing .
- Combination Therapies : Co-administration with ENaC inhibitors (e.g., amiloride) or CFTR modulators may amplify ASL hydration .
Q. How can contradictory data between in vitro and clinical outcomes be reconciled?
In vitro models (e.g., HBE cells) lack the complex mucus dynamics and inflammation of CF airways. To bridge this gap:
- Advanced 3D Models : Air-liquid interface cultures with Pseudomonas aeruginosa biofilms better mimic in vivo conditions .
- Imaging Techniques : Confocal microscopy quantifies ASL height and ciliary beat frequency in real time .
- Translational Biomarkers : DTPA clearance rates and sputum IL-8 levels correlate preclinical and clinical efficacy .
Q. What lessons from this compound’s development inform future CF therapeutic design?
- Early Pharmacodynamic Profiling : Preclinical studies must assess receptor desensitization and pharmacokinetics in disease-relevant models .
- Adaptive Trial Designs : Seamless Phase II/III trials with biomarker enrichment (e.g., FEV₁ >80%) can optimize patient selection .
- Post-Hoc Analysis Rigor : Secondary endpoints (e.g., exacerbation rates) may reveal efficacy masked by primary endpoint limitations .
Properties
Key on ui mechanism of action |
Denufosol tetrasodium is a selective P2Y2 agonist designed to enhance the lung's innate mucosal hydration and mucociliary clearance mechanisms by activating an alternative ion channel that acts in the same way as the defective ion channel in moving salt and water to the surface of the airways. Based on pre-clinical and clinical work, denufosol has several pharmacological actions contributing to its mechanism of action: hydration of the airways by stimulating chloride and liquid secretions on the epithelial cell surface; inhibition of epithelial sodium absorption; enhancement of ciliary beat frequency; and stimulation of mucin secretion. This unique mechanism of action represents a differentiated approach relative to other approved CF products and may be important in intervening in the early clinical course of CF lung disease. |
---|---|
CAS No. |
211448-85-0 |
Molecular Formula |
C18H27N5O21P4 |
Molecular Weight |
773.3 g/mol |
IUPAC Name |
[[(2R,3S,5R)-5-(4-amino-2-oxopyrimidin-1-yl)-3-hydroxyoxolan-2-yl]methoxy-hydroxyphosphoryl] [[[(2R,3S,4R,5R)-5-(2,4-dioxopyrimidin-1-yl)-3,4-dihydroxyoxolan-2-yl]methoxy-hydroxyphosphoryl]oxy-hydroxyphosphoryl] hydrogen phosphate |
InChI |
InChI=1S/C18H27N5O21P4/c19-11-1-3-22(17(28)20-11)13-5-8(24)9(40-13)6-38-45(30,31)42-47(34,35)44-48(36,37)43-46(32,33)39-7-10-14(26)15(27)16(41-10)23-4-2-12(25)21-18(23)29/h1-4,8-10,13-16,24,26-27H,5-7H2,(H,30,31)(H,32,33)(H,34,35)(H,36,37)(H2,19,20,28)(H,21,25,29)/t8-,9+,10+,13+,14+,15+,16+/m0/s1 |
InChI Key |
FPNPSEMJLALQSA-MIYUEGBISA-N |
SMILES |
C1C(C(OC1N2C=CC(=NC2=O)N)COP(=O)(O)OP(=O)(O)OP(=O)(O)OP(=O)(O)OCC3C(C(C(O3)N4C=CC(=O)NC4=O)O)O)O |
Isomeric SMILES |
C1[C@@H]([C@H](O[C@H]1N2C=CC(=NC2=O)N)COP(=O)(O)OP(=O)(O)OP(=O)(O)OP(=O)(O)OC[C@@H]3[C@H]([C@H]([C@@H](O3)N4C=CC(=O)NC4=O)O)O)O |
Canonical SMILES |
C1C(C(OC1N2C=CC(=NC2=O)N)COP(=O)(O)OP(=O)(O)OP(=O)(O)OP(=O)(O)OCC3C(C(C(O3)N4C=CC(=O)NC4=O)O)O)O |
Key on ui other cas no. |
211448-85-0 |
Synonyms |
denufosol tetrasodium INS 37217 INS37217 P(1)-(uridine 5')-P(4)- (2'-deoxycytidine 5')tetraphosphate, tetrasodium salt |
Origin of Product |
United States |
Retrosynthesis Analysis
AI-Powered Synthesis Planning: Our tool employs the Template_relevance Pistachio, Template_relevance Bkms_metabolic, Template_relevance Pistachio_ringbreaker, Template_relevance Reaxys, Template_relevance Reaxys_biocatalysis model, leveraging a vast database of chemical reactions to predict feasible synthetic routes.
One-Step Synthesis Focus: Specifically designed for one-step synthesis, it provides concise and direct routes for your target compounds, streamlining the synthesis process.
Accurate Predictions: Utilizing the extensive PISTACHIO, BKMS_METABOLIC, PISTACHIO_RINGBREAKER, REAXYS, REAXYS_BIOCATALYSIS database, our tool offers high-accuracy predictions, reflecting the latest in chemical research and data.
Strategy Settings
Precursor scoring | Relevance Heuristic |
---|---|
Min. plausibility | 0.01 |
Model | Template_relevance |
Template Set | Pistachio/Bkms_metabolic/Pistachio_ringbreaker/Reaxys/Reaxys_biocatalysis |
Top-N result to add to graph | 6 |
Feasible Synthetic Routes
Disclaimer and Information on In-Vitro Research Products
Please be aware that all articles and product information presented on BenchChem are intended solely for informational purposes. The products available for purchase on BenchChem are specifically designed for in-vitro studies, which are conducted outside of living organisms. In-vitro studies, derived from the Latin term "in glass," involve experiments performed in controlled laboratory settings using cells or tissues. It is important to note that these products are not categorized as medicines or drugs, and they have not received approval from the FDA for the prevention, treatment, or cure of any medical condition, ailment, or disease. We must emphasize that any form of bodily introduction of these products into humans or animals is strictly prohibited by law. It is essential to adhere to these guidelines to ensure compliance with legal and ethical standards in research and experimentation.