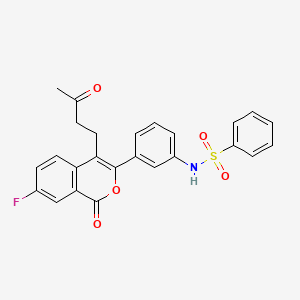
Pde4-IN-6
- Click on QUICK INQUIRY to receive a quote from our team of experts.
- With the quality product at a COMPETITIVE price, you can focus more on your research.
Overview
Description
Pde4-IN-6 is a compound that belongs to the class of phosphodiesterase 4 inhibitors. Phosphodiesterase 4 inhibitors are a family of enzymes that specifically promote the hydrolysis and degradation of cyclic adenosine monophosphate. The inhibition of phosphodiesterase 4 enzymes has been widely investigated as a possible alternative strategy for the treatment of a variety of respiratory diseases, including chronic obstructive pulmonary disease and asthma, as well as psoriasis and other autoimmune disorders .
Preparation Methods
The synthesis of phosphodiesterase 4 inhibitors, including Pde4-IN-6, involves several steps. The synthetic routes and reaction conditions can vary, but typically involve the use of specific reagents and catalysts to achieve the desired chemical structure. Industrial production methods for phosphodiesterase 4 inhibitors often involve large-scale synthesis using optimized reaction conditions to ensure high yield and purity .
Chemical Reactions Analysis
Pde4-IN-6 undergoes various types of chemical reactions, including oxidation, reduction, and substitution reactions. Common reagents and conditions used in these reactions include oxidizing agents, reducing agents, and nucleophiles. The major products formed from these reactions depend on the specific reaction conditions and reagents used .
Scientific Research Applications
Background on Phosphodiesterase 4
Phosphodiesterase 4 (PDE4) is an enzyme that hydrolyzes cyclic adenosine monophosphate (cAMP), a critical intracellular signaling molecule involved in numerous physiological processes. Inhibition of PDE4 leads to increased levels of cAMP, which can modulate inflammatory responses, immune cell activation, and other cellular functions. This mechanism has made PDE4 an attractive target for drug development, especially for conditions characterized by excessive inflammation.
Anti-Inflammatory Effects
PDE4-IN-6 has been studied extensively for its anti-inflammatory properties. Research indicates that PDE4 inhibitors can effectively suppress inflammatory responses in various models:
- Asthma and Chronic Obstructive Pulmonary Disease (COPD): Inhibitors like this compound have shown efficacy in reducing airway inflammation and improving lung function in preclinical models of asthma and COPD . Clinical trials have demonstrated significant reductions in inflammation-related biomarkers following treatment with PDE4 inhibitors .
- Psoriasis: The compound has been identified as a potential treatment for psoriasis, where it may help control symptoms by modulating immune responses and reducing skin inflammation . In silico studies have highlighted its potential as a lead compound in drug development for this condition.
Immunomodulatory Effects
This compound also exhibits immunomodulatory effects that can benefit conditions like rheumatoid arthritis and inflammatory bowel disease (IBD). By inhibiting PDE4, the compound enhances cAMP signaling pathways that downregulate pro-inflammatory cytokines .
Cognitive Enhancement
Recent studies suggest that PDE4 inhibitors may have cognitive-enhancing effects, potentially benefiting conditions such as Alzheimer's disease and depression. The modulation of cAMP levels is linked to improved synaptic plasticity and memory function .
Case Study 1: CHF 6001 in Asthma Patients
In a double-blind study involving asthmatic patients, CHF 6001 (a potent PDE4 inhibitor) demonstrated significant efficacy in reducing allergen-induced late asthmatic responses. Patients receiving inhaled doses showed improved lung function and reduced inflammation markers .
Case Study 2: Roflumilast in B-cell Malignancies
A pilot study explored the use of Roflumilast, another PDE4 inhibitor, in patients with advanced B-cell malignancies. The treatment was well-tolerated and resulted in significant tumor burden reduction, highlighting the potential of PDE4 inhibition in cancer therapy .
Data Tables
Application Area | Compound | Mechanism of Action | Key Findings |
---|---|---|---|
Asthma | This compound | Inhibition of PDE4 leads to increased cAMP | Significant reduction in airway inflammation |
COPD | CHF 6001 | Anti-inflammatory effects | Improved lung function and reduced biomarkers |
Psoriasis | Novel Inhibitors | Modulation of immune response | Effective in reducing skin lesions |
Cognitive Disorders | Roflumilast | Enhances synaptic plasticity | Potential improvement in memory functions |
Mechanism of Action
The mechanism of action of Pde4-IN-6 involves the inhibition of phosphodiesterase 4 enzymes, leading to an increase in cyclic adenosine monophosphate levels. This increase in cyclic adenosine monophosphate levels results in the activation of protein kinase A and the subsequent regulation of various genes and proteins involved in inflammation and other cellular processes. The molecular targets and pathways involved in the mechanism of action of this compound include the cyclic adenosine monophosphate response element-binding protein and other signaling molecules .
Comparison with Similar Compounds
Pde4-IN-6 is similar to other phosphodiesterase 4 inhibitors, such as roflumilast, apremilast, and crisaborole. this compound has unique structural features that contribute to its high selectivity and potency as a phosphodiesterase 4 inhibitor. The comparison with other similar compounds highlights the uniqueness of this compound in terms of its binding properties, inhibitory efficacy, and potential as a therapeutic agent .
Biological Activity
Pde4-IN-6 is a selective inhibitor of phosphodiesterase 4 (PDE4), a key enzyme involved in the hydrolysis of cyclic adenosine monophosphate (cAMP). This compound has garnered attention for its potential therapeutic applications, particularly in inflammatory diseases and conditions involving immune modulation. This article delves into the biological activity of this compound, exploring its mechanisms, effects on various cell types, and implications for clinical use.
Overview of PDE4 and Its Inhibitors
Phosphodiesterases (PDEs) are a family of enzymes responsible for the degradation of cyclic nucleotides such as cAMP and cyclic guanosine monophosphate (cGMP). The PDE4 subfamily is particularly significant due to its extensive distribution across various tissues, including the brain, lungs, and immune cells. It plays crucial roles in regulating inflammatory responses, neuronal signaling, and immune cell function .
PDE4 inhibitors, including this compound, have been shown to increase intracellular cAMP levels, leading to various biological effects such as:
- Anti-inflammatory responses : Reduction in pro-inflammatory cytokines.
- Immunomodulation : Altered T cell activation and function.
- Neuroprotection : Potential benefits in neurodegenerative diseases.
This compound selectively inhibits PDE4 activity, which results in elevated cAMP concentrations within cells. This elevation leads to downstream effects on various signaling pathways:
- Inhibition of pro-inflammatory cytokines : Increased cAMP inhibits the release of TNF-α and IL-6 from macrophages and other immune cells .
- Regulation of T cell responses : PDE4 inhibition can modulate T cell receptor signaling, enhancing IL-2 production while suppressing other pro-inflammatory cytokines like IFN-γ .
- Effects on smooth muscle cells : In vascular smooth muscle cells, increased cAMP can lead to relaxation and reduced proliferation, highlighting potential cardiovascular benefits .
Research Findings and Case Studies
Recent studies have investigated the efficacy of this compound in various models:
-
Inflammatory Disease Models :
- In murine models of asthma and chronic obstructive pulmonary disease (COPD), this compound demonstrated significant reductions in airway hyperresponsiveness and inflammation markers .
- A study involving human macrophages showed that treatment with this compound resulted in decreased TNF-α release upon lipopolysaccharide (LPS) stimulation .
- Cancer Research :
- Neurodegenerative Diseases :
Data Summary
The following table summarizes key findings from recent studies on this compound:
Study Focus | Model Type | Key Findings |
---|---|---|
Inflammation | Murine asthma model | Reduced airway hyperresponsiveness; decreased inflammatory markers |
Immune Response | Human macrophages | Decreased TNF-α release upon LPS stimulation |
Cancer Therapy | B cell lymphoma | Enhanced glucocorticoid sensitivity; reduced tumor burden |
Cognitive Function | Alzheimer’s model | Improved synaptic plasticity; enhanced cognitive performance |
Properties
Molecular Formula |
C25H20FNO5S |
---|---|
Molecular Weight |
465.5 g/mol |
IUPAC Name |
N-[3-[7-fluoro-1-oxo-4-(3-oxobutyl)isochromen-3-yl]phenyl]benzenesulfonamide |
InChI |
InChI=1S/C25H20FNO5S/c1-16(28)10-12-22-21-13-11-18(26)15-23(21)25(29)32-24(22)17-6-5-7-19(14-17)27-33(30,31)20-8-3-2-4-9-20/h2-9,11,13-15,27H,10,12H2,1H3 |
InChI Key |
QOQQISZJYVVAJT-UHFFFAOYSA-N |
Canonical SMILES |
CC(=O)CCC1=C(OC(=O)C2=C1C=CC(=C2)F)C3=CC(=CC=C3)NS(=O)(=O)C4=CC=CC=C4 |
Origin of Product |
United States |
Disclaimer and Information on In-Vitro Research Products
Please be aware that all articles and product information presented on BenchChem are intended solely for informational purposes. The products available for purchase on BenchChem are specifically designed for in-vitro studies, which are conducted outside of living organisms. In-vitro studies, derived from the Latin term "in glass," involve experiments performed in controlled laboratory settings using cells or tissues. It is important to note that these products are not categorized as medicines or drugs, and they have not received approval from the FDA for the prevention, treatment, or cure of any medical condition, ailment, or disease. We must emphasize that any form of bodily introduction of these products into humans or animals is strictly prohibited by law. It is essential to adhere to these guidelines to ensure compliance with legal and ethical standards in research and experimentation.