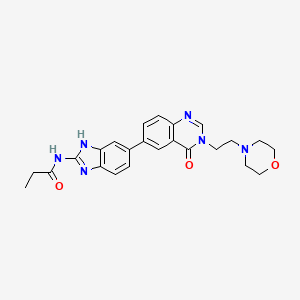
Aurora A inhibitor 2
- Click on QUICK INQUIRY to receive a quote from our team of experts.
- With the quality product at a COMPETITIVE price, you can focus more on your research.
Overview
Description
Aurora A inhibitor 2 is a small molecule compound designed to selectively inhibit Aurora kinase A, a serine/threonine kinase crucial for cell division and mitosis. Aurora kinase A is often overexpressed in various cancers, making it a significant target for cancer therapy . The inhibition of Aurora kinase A can disrupt mitotic processes, leading to cell cycle arrest and apoptosis in cancer cells .
Preparation Methods
Synthetic Routes and Reaction Conditions: The synthesis of Aurora A inhibitor 2 typically involves multiple steps, including the formation of key intermediates and final coupling reactions. One common synthetic route includes:
Formation of the Core Structure: This involves the construction of the core heterocyclic structure through cyclization reactions.
Functional Group Modifications: Introduction of functional groups such as amines, hydroxyls, or halogens to enhance binding affinity and selectivity.
Final Coupling: The final step often involves coupling the core structure with specific side chains or substituents to achieve the desired inhibitory activity.
Industrial Production Methods: Industrial production of this compound may involve large-scale synthesis using optimized reaction conditions to ensure high yield and purity. This includes:
Batch Processing: Utilizing batch reactors for controlled synthesis.
Purification: Employing techniques such as crystallization, chromatography, and recrystallization to purify the final product.
Chemical Reactions Analysis
Types of Reactions: Aurora A inhibitor 2 can undergo various chemical reactions, including:
Oxidation: Introduction of oxygen atoms to form oxides or hydroxides.
Reduction: Removal of oxygen atoms or addition of hydrogen atoms to form reduced compounds.
Substitution: Replacement of functional groups with other groups to modify the compound’s properties.
Common Reagents and Conditions:
Oxidizing Agents: Hydrogen peroxide, potassium permanganate.
Reducing Agents: Sodium borohydride, lithium aluminum hydride.
Substitution Reagents: Halogens, alkylating agents.
Major Products: The major products formed from these reactions include various derivatives of this compound with modified functional groups, enhancing its pharmacological properties .
Scientific Research Applications
Aurora A inhibitor 2 has a wide range of scientific research applications, including:
Cancer Research: Used to study the role of Aurora kinase A in cancer cell proliferation and tumor growth.
Cell Cycle Studies: Employed to investigate the mechanisms of mitosis and cell division, providing insights into the regulation of the cell cycle.
Drug Development: Serves as a lead compound for developing new anticancer therapies targeting Aurora kinase A.
Biological Research: Utilized in studies exploring the interaction of Aurora kinase A with other cellular proteins and pathways.
Mechanism of Action
Aurora A inhibitor 2 exerts its effects by binding to the active site of Aurora kinase A, preventing its phosphorylation and activation. This inhibition disrupts the kinase’s ability to regulate mitosis, leading to cell cycle arrest and apoptosis in cancer cells . The molecular targets and pathways involved include:
Phosphorylation Inhibition: Blocking the phosphorylation of key substrates involved in mitosis.
Disruption of Spindle Assembly: Interfering with the formation of the mitotic spindle, essential for chromosome segregation.
Induction of Apoptosis: Triggering programmed cell death in cancer cells through various apoptotic pathways.
Comparison with Similar Compounds
Aurora A inhibitor 2 can be compared with other similar compounds, such as:
MK-8745: Another Aurora kinase A inhibitor with distinct binding affinities and selectivity profiles.
TC-A2317: A compound that targets both Aurora kinase A and B, offering broader inhibitory effects.
Uniqueness: this compound stands out due to its high selectivity for Aurora kinase A, minimizing off-target effects and enhancing its therapeutic potential .
Properties
Molecular Formula |
C24H26N6O3 |
---|---|
Molecular Weight |
446.5 g/mol |
IUPAC Name |
N-[6-[3-(2-morpholin-4-ylethyl)-4-oxoquinazolin-6-yl]-1H-benzimidazol-2-yl]propanamide |
InChI |
InChI=1S/C24H26N6O3/c1-2-22(31)28-24-26-20-6-4-17(14-21(20)27-24)16-3-5-19-18(13-16)23(32)30(15-25-19)8-7-29-9-11-33-12-10-29/h3-6,13-15H,2,7-12H2,1H3,(H2,26,27,28,31) |
InChI Key |
WKBADCPZJIWSRC-UHFFFAOYSA-N |
Canonical SMILES |
CCC(=O)NC1=NC2=C(N1)C=C(C=C2)C3=CC4=C(C=C3)N=CN(C4=O)CCN5CCOCC5 |
Origin of Product |
United States |
Disclaimer and Information on In-Vitro Research Products
Please be aware that all articles and product information presented on BenchChem are intended solely for informational purposes. The products available for purchase on BenchChem are specifically designed for in-vitro studies, which are conducted outside of living organisms. In-vitro studies, derived from the Latin term "in glass," involve experiments performed in controlled laboratory settings using cells or tissues. It is important to note that these products are not categorized as medicines or drugs, and they have not received approval from the FDA for the prevention, treatment, or cure of any medical condition, ailment, or disease. We must emphasize that any form of bodily introduction of these products into humans or animals is strictly prohibited by law. It is essential to adhere to these guidelines to ensure compliance with legal and ethical standards in research and experimentation.