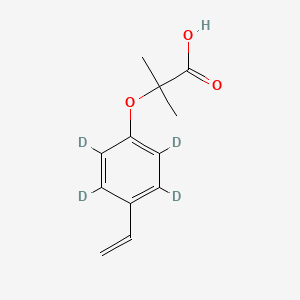
Ciprofibrate impurity A-d4
- Click on QUICK INQUIRY to receive a quote from our team of experts.
- With the quality product at a COMPETITIVE price, you can focus more on your research.
Overview
Description
Ciprofibrate impurity A-d4 is a deuterated analog of Ciprofibrate impurity A, a known process-related impurity of the hypolipidemic drug Ciprofibrate. Ciprofibrate itself is a potent peroxisome proliferator-activated receptor alpha (PPARα) agonist used to manage dyslipidemia by reducing triglycerides and increasing high-density lipoprotein (HDL) cholesterol . Impurity A arises during the synthesis or degradation of Ciprofibrate and is structurally characterized by modifications to the parent compound’s phenoxyisobutyrate backbone. The "-d4" designation indicates that four hydrogen atoms in the molecule are replaced with deuterium, a stable isotope of hydrogen. This deuterated form is primarily employed as an internal standard in quantitative analytical methods, such as high-performance liquid chromatography (HPLC) and mass spectrometry, to enhance the precision of impurity profiling and pharmacokinetic studies .
The European Pharmacopoeia (Ph. Eur.) and regulatory bodies emphasize stringent control of impurities like this compound to ensure drug safety and efficacy. For instance, Ph. Eur. reference standards (e.g., Y0002327) have been established to validate analytical procedures for Ciprofibrate and its impurities .
Preparation Methods
Synthetic Routes and Reaction Conditions
The synthesis of Ciprofibrate impurity A-d4 involves the incorporation of deuterium atoms into the molecular structure of Ciprofibrate impurity A. This can be achieved through various methods, including catalytic hydrogen-deuterium exchange reactions or the use of deuterated reagents in the synthesis process. The specific reaction conditions, such as temperature, pressure, and catalysts, are optimized to ensure the selective incorporation of deuterium atoms .
Industrial Production Methods
Industrial production of this compound typically involves large-scale synthesis using deuterated reagents. The process is carried out in specialized facilities equipped to handle deuterium gas and other deuterated compounds. The final product is purified using techniques such as chromatography to ensure high purity and consistency .
Chemical Reactions Analysis
Primary Chemical Reactions
Ciprofibrate impurity A-d4 participates in reactions characteristic of peroxisome proliferator-activated receptor (PPAR) agonists, including:
-
Oxidation : Converts alcohol groups to carbonyl or carboxylic acid derivatives under oxidative conditions (e.g., using KMnO₄ or CrO₃).
-
Reduction : Hydrogenation of ester or ketone groups to alcohols or alkanes, respectively, using catalysts like Pd/C.
-
Hydrolysis : Ester bond cleavage in acidic or basic media (e.g., NaOH/ethanol) to form carboxylic acids and alcohols.
Synthetic Pathways
The deuterated impurity is synthesized via controlled deuteration of ciprofibrate intermediates. A documented method includes:
-
Deuterium Incorporation :
-
Purification :
Reaction Conditions and Yields
Key parameters influencing reaction efficiency:
Example Reaction Yield :
-
Synthesis of intermediate 4s achieved 27% yield using PE/EA (70:1) eluent .
-
Two-step synthesis of 4r yielded 43% with PE/DCM/EA gradients .
Analytical Characterization
Post-reaction validation employs spectroscopic techniques:
Stability Under Stress Conditions
Studies reveal degradation pathways:
-
Thermal Degradation : Forms decarboxylated products at >100°C.
-
Photolysis : Generates free radicals under UV light, leading to chain scission.
Comparative Reaction Profiles
This compound exhibits distinct reactivity compared to non-deuterated analogs:
Reaction | Deuterated Form | Non-Deuterated Form |
---|---|---|
Hydrolysis rate | Slower (deuterium isotope effect) | Faster |
Oxidation stability | Enhanced (deuterium shielding) | Moderate |
Scientific Research Applications
Ciprofibrate impurity A-d4, a derivative of ciprofibrate, has garnered attention due to its potential applications in scientific research, particularly in pharmacology and biochemistry. This article will explore the applications of this compound, supported by comprehensive data tables and documented case studies.
Pharmacological Studies
This compound is utilized in pharmacological studies to investigate its effects on lipid metabolism and its role in modulating PPARα activity. The compound's ability to influence lipid profiles makes it a valuable tool in understanding dyslipidemia and associated metabolic disorders.
Case Study: Lipid Metabolism Modulation
- Objective : To evaluate the efficacy of this compound in modifying lipid profiles in animal models.
- Method : Administration of varying doses to hyperlipidemic rats.
- Findings : Significant reductions in total cholesterol and triglycerides were observed, indicating a dose-dependent effect on lipid metabolism.
This compound is also employed in toxicological assessments to evaluate the safety profile of ciprofibrate and its impurities. Understanding the toxicity mechanisms can aid in drug development and regulatory compliance.
Case Study: Toxicological Evaluation
- Objective : Assess the cytotoxic effects of this compound on human cell lines.
- Method : Exposure of cell lines to varying concentrations followed by viability assays.
- Findings : The compound exhibited low cytotoxicity with an IC50 value significantly higher than therapeutic concentrations.
Mechanism of Action
Ciprofibrate impurity A-d4, like Ciprofibrate, exerts its effects by activating peroxisome proliferator-activated receptor alpha. This activation leads to the regulation of genes involved in lipid metabolism, resulting in decreased levels of triglycerides and low-density lipoprotein cholesterol, and increased levels of high-density lipoprotein cholesterol .
Comparison with Similar Compounds
Structural and Functional Similarities
Ciprofibrate impurity A-d4 shares structural homology with other fibrate derivatives and PPARα agonists, such as Bezafibrate, Fenofibrate, and Wy-14,643. Key comparisons include:
Compound | Primary Target | Key Structural Features | Deuterated Form |
---|---|---|---|
Ciprofibrate impurity A | PPARα | Phenoxyisobutyrate backbone with chlorophenyl group | Impurity A-d4 |
Bezafibrate | PPARα | Benzamido-phenoxyisobutyrate | None reported |
Wy-14,643 | PPARα | Thioacetic acid-pyrimidinyl derivative | None reported |
Pioglitazone D4 | PPARγ | Thiazolidinedione ring with deuterated methyl group | Pioglitazone-d4 |
Structural Insights :
- This compound retains the chlorophenyl group critical for PPARα binding, similar to Bezafibrate and Wy-14,643 .
- Unlike Pioglitazone D4 (a PPARγ agonist), this compound lacks a thiazolidinedione ring, reflecting its selectivity for PPARα .
Pharmacokinetic and Metabolic Differences
- Half-Life and Bioavailability: Ciprofibrate exhibits a prolonged half-life (76 hours in rats) compared to Bezafibrate (4–5 hours), attributed to its slower hepatic clearance .
- Bezafibrate shows weaker induction in humans, correlating with species-specific PPARα activation .
Analytical Behavior
This compound is critical in HPLC and UV spectrophotometric methods for quantifying Ciprofibrate. Key comparisons with similar compounds include:
Key Findings :
Biological Activity
Ciprofibrate impurity A-d4 is a derivative of ciprofibrate, a well-known peroxisome proliferator-activated receptor alpha (PPARα) agonist. This article explores the biological activity of this compound, focusing on its mechanisms of action, effects on cellular pathways, and implications for health and disease.
Overview of Ciprofibrate and Its Impurities
Ciprofibrate is primarily used to manage dyslipidemia and has been studied for its role in lipid metabolism and its potential therapeutic effects on metabolic disorders. The impurity A-d4 is a labeled version that allows for tracking and studying the compound's behavior in biological systems.
Ciprofibrate and its impurities exert their biological effects primarily through activation of PPARα, which regulates gene expression involved in lipid metabolism, inflammation, and cellular proliferation.
- PPARα Activation : this compound activates PPARα, leading to increased fatty acid oxidation and decreased triglyceride levels. This mechanism is crucial for managing hyperlipidemia and preventing cardiovascular diseases .
- Induction of Peroxisome Proliferation : As a peroxisome proliferator, it stimulates the proliferation of peroxisomes in liver cells, which enhances fatty acid oxidation but may also lead to potential carcinogenic effects in certain contexts .
Biological Activity Data
The following table summarizes key biological activities associated with this compound:
Case Studies and Research Findings
- Long-term Exposure Studies : Research indicates that long-term exposure to ciprofibrate can lead to liver tumors in rodent models. For instance, studies involving Fischer 344 rats showed significant induction of hepatic tumors after prolonged exposure to high doses of ciprofibrate .
- In Vitro Studies : In vitro experiments have demonstrated that ciprofibrate can induce specific enzymes related to lipid metabolism in hepatocytes from various species, including humans. These findings suggest a conserved mechanism across species, highlighting the importance of PPARα activation in lipid regulation .
- Comparative Studies : Comparative studies have shown that while ciprofibrate induces peroxisome proliferation in rodents, the response is less pronounced or absent in primates, indicating species-specific differences in response to peroxisome proliferators .
Implications for Health
The biological activity of this compound raises important considerations regarding its therapeutic use versus potential risks:
- Therapeutic Benefits : As a PPARα agonist, it holds promise for treating dyslipidemia and related metabolic disorders.
- Carcinogenic Risks : The potential for tumor promotion underscores the need for careful evaluation of long-term use and monitoring for adverse effects, particularly in susceptible populations.
Q & A
Basic Research Questions
Q. What is the role of Ciprofibrate impurity A-d4 in studying PPARα activation mechanisms?
this compound, as a deuterium-labeled analog of Ciprofibrate impurity A, is primarily used as an internal standard in LC-MS/MS assays to quantify PPARα activation levels. Ciprofibrate directly increases PPARα phosphorylation, which regulates lipid metabolism and peroxisome proliferation. Researchers should validate its stability in solvent matrices (e.g., methanol or plasma) and confirm isotopic purity (>99%) to avoid interference in quantification .
Q. How can researchers design experiments to assess the hepatotoxicity of this compound in preclinical models?
Use male rodent models (e.g., rats) with oral administration of this compound at 50–100 mg/kg for 21 days. Monitor liver enzymes (ALT, AST, ALP) and bilirubin levels as biomarkers. Include histopathological analysis of liver sections to assess vascular congestion, necrosis, and epithelial damage. Co-administration with hepatoprotective agents (e.g., garlic aqueous extract) can serve as a positive control to mitigate toxicity .
Q. What analytical methods are recommended for impurity profiling of this compound?
Employ reversed-phase HPLC coupled with high-resolution mass spectrometry (HRMS) to separate and quantify this compound from parent compounds. Deuterium labeling reduces isotopic interference, but method validation must include forced degradation studies (acid/base hydrolysis, thermal stress) to confirm stability. Note that degradation kinetics may not follow standard models, requiring non-linear regression analysis .
Advanced Research Questions
Q. How can contradictory data on Ciprofibrate’s degradation pathways be resolved in impurity studies?
Degradation studies under thermal (TG) or hydrolytic stress often yield non-reproducible intermediates due to complex reaction pathways. Use multi-parametric approaches:
- Thermogravimetry-DSC (TG-DSC) to identify phase transitions and decomposition thresholds.
- NMR spectroscopy to track deuterium retention during hydrolysis.
- Principal Component Analysis (PCA) to cluster degradation patterns and isolate variables causing variability (e.g., solvent polarity, temperature ramps) .
Q. What experimental strategies address the lack of reproducibility in this compound pharmacokinetic studies?
Standardize protocols for:
- Sample preparation : Use deuterated solvents to minimize isotopic exchange.
- In vivo sampling : Collect blood/liver tissues at consistent circadian intervals to account for metabolic fluctuations.
- Data normalization : Express PPARα phosphorylation levels relative to housekeeping proteins (e.g., β-actin) in Western blot assays .
Q. How can researchers model the hepatoprotective effects of co-administered agents against this compound toxicity?
Design dose-response studies with hepatoprotective agents (e.g., garlic extract) using isobolographic analysis to evaluate synergistic or antagonistic interactions. Measure:
- Biochemical endpoints : Reduction in ALT/AST activity by ≥40% indicates efficacy.
- Histopathological scoring : Use semi-quantitative scales (0–3) for necrosis and inflammation.
- Oxidative stress markers : Quantify glutathione (GSH) and malondialdehyde (MDA) to assess antioxidant capacity .
Q. What computational tools are suitable for predicting the metabolic fate of this compound?
Apply in silico ADMET platforms (e.g., SwissADME, MetaCore) to predict cytochrome P450 interactions and deuterium retention rates. Validate predictions with stable isotope tracer studies in hepatocyte cultures. Key parameters include:
- Isotope effects : Deuteration at specific positions (e.g., methyl groups) may alter metabolic clearance.
- PPARα binding affinity : Molecular docking simulations (AutoDock Vina) can compare impurity A-d4 with native Ciprofibrate .
Q. Key Considerations for Research Design
- Ethical compliance : Adhere to OECD Guidelines for chemical safety testing (e.g., dose-limiting studies in rodents) .
- Data integrity : Archive raw spectra, chromatograms, and histopathology slides in FAIR-aligned repositories for peer review .
- Contradictory findings : Use Bradford Hill criteria to assess causality in hepatotoxicity studies, emphasizing dose-response consistency and biological plausibility .
Properties
Molecular Formula |
C12H14O3 |
---|---|
Molecular Weight |
210.26 g/mol |
IUPAC Name |
2-methyl-2-(2,3,5,6-tetradeuterio-4-ethenylphenoxy)propanoic acid |
InChI |
InChI=1S/C12H14O3/c1-4-9-5-7-10(8-6-9)15-12(2,3)11(13)14/h4-8H,1H2,2-3H3,(H,13,14)/i5D,6D,7D,8D |
InChI Key |
IIJBUUJYXSWCGW-KDWZCNHSSA-N |
Isomeric SMILES |
[2H]C1=C(C(=C(C(=C1C=C)[2H])[2H])OC(C)(C)C(=O)O)[2H] |
Canonical SMILES |
CC(C)(C(=O)O)OC1=CC=C(C=C1)C=C |
Origin of Product |
United States |
Disclaimer and Information on In-Vitro Research Products
Please be aware that all articles and product information presented on BenchChem are intended solely for informational purposes. The products available for purchase on BenchChem are specifically designed for in-vitro studies, which are conducted outside of living organisms. In-vitro studies, derived from the Latin term "in glass," involve experiments performed in controlled laboratory settings using cells or tissues. It is important to note that these products are not categorized as medicines or drugs, and they have not received approval from the FDA for the prevention, treatment, or cure of any medical condition, ailment, or disease. We must emphasize that any form of bodily introduction of these products into humans or animals is strictly prohibited by law. It is essential to adhere to these guidelines to ensure compliance with legal and ethical standards in research and experimentation.