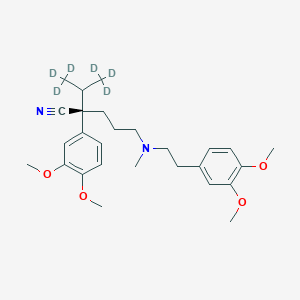
(R)-(+)-Verapamil-d6
- Click on QUICK INQUIRY to receive a quote from our team of experts.
- With the quality product at a COMPETITIVE price, you can focus more on your research.
Overview
Description
®-(+)-Verapamil-d6 is a deuterated form of the well-known calcium channel blocker, Verapamil. This compound is specifically labeled with deuterium, which is a stable isotope of hydrogen. The presence of deuterium atoms in place of hydrogen atoms can significantly alter the pharmacokinetic properties of the compound, making it a valuable tool in scientific research, particularly in the fields of pharmacology and drug metabolism studies.
Preparation Methods
Synthetic Routes and Reaction Conditions
The synthesis of ®-(+)-Verapamil-d6 involves the incorporation of deuterium atoms into the Verapamil molecule. This can be achieved through various synthetic routes, including:
-
Hydrogen-Deuterium Exchange Reactions: : This method involves the replacement of hydrogen atoms with deuterium atoms in the presence of a deuterium source such as deuterium oxide (D2O) or deuterated solvents. The reaction conditions typically involve elevated temperatures and the use of catalysts to facilitate the exchange process.
-
Deuterated Reagents: : Another approach is to use deuterated reagents in the synthesis of Verapamil. For example, deuterated benzyl chloride can be used in the alkylation step to introduce deuterium atoms into the final product.
Industrial Production Methods
The industrial production of ®-(+)-Verapamil-d6 follows similar principles as the laboratory synthesis but on a larger scale. The process involves the use of high-purity deuterium sources and optimized reaction conditions to ensure the efficient incorporation of deuterium atoms. The final product is then purified using standard techniques such as crystallization, distillation, or chromatography to achieve the desired level of deuteration and purity.
Chemical Reactions Analysis
Types of Reactions
®-(+)-Verapamil-d6 undergoes various chemical reactions, including:
Oxidation: The compound can be oxidized to form corresponding N-oxide derivatives.
Reduction: Reduction reactions can convert the compound into its reduced forms.
Substitution: The aromatic rings in the compound can undergo electrophilic substitution reactions.
Common Reagents and Conditions
Oxidation: Common oxidizing agents include hydrogen peroxide (H2O2) and potassium permanganate (KMnO4).
Reduction: Reducing agents such as lithium aluminum hydride (LiAlH4) and sodium borohydride (NaBH4) are commonly used.
Substitution: Electrophilic substitution reactions often involve reagents like bromine (Br2) or nitric acid (HNO3).
Major Products
The major products formed from these reactions depend on the specific reaction conditions and reagents used. For example, oxidation can yield N-oxide derivatives, while substitution reactions can introduce various functional groups into the aromatic rings.
Scientific Research Applications
®-(+)-Verapamil-d6 has several scientific research applications, including:
Pharmacokinetic Studies: The deuterium labeling allows for the tracking of the compound in biological systems, providing valuable data on its absorption, distribution, metabolism, and excretion.
Drug Metabolism: The compound is used to study the metabolic pathways and identify the metabolites formed in the body.
Isotope Effects: Researchers use ®-(+)-Verapamil-d6 to study the kinetic isotope effects, which can provide insights into the reaction mechanisms and the role of hydrogen atoms in biological processes.
Analytical Chemistry: The compound serves as an internal standard in mass spectrometry and other analytical techniques due to its distinct isotopic signature.
Mechanism of Action
®-(+)-Verapamil-d6, like its non-deuterated counterpart, acts as a calcium channel blocker. It inhibits the influx of calcium ions through L-type calcium channels in the cell membrane. This inhibition leads to the relaxation of vascular smooth muscle, resulting in vasodilation and a decrease in blood pressure. The compound also affects cardiac muscle cells, reducing heart rate and contractility. The molecular targets include the alpha-1 subunit of the L-type calcium channels, and the pathways involved are related to calcium signaling and muscle contraction.
Comparison with Similar Compounds
Similar Compounds
Verapamil: The non-deuterated form of ®-(+)-Verapamil-d6, widely used as a calcium channel blocker.
Diltiazem: Another calcium channel blocker with a similar mechanism of action but different chemical structure.
Amlodipine: A dihydropyridine calcium channel blocker with a longer duration of action.
Uniqueness
®-(+)-Verapamil-d6 is unique due to its deuterium labeling, which provides distinct advantages in pharmacokinetic and metabolic studies. The presence of deuterium atoms can lead to a slower metabolic rate, potentially resulting in prolonged drug action and reduced formation of toxic metabolites. This makes ®-(+)-Verapamil-d6 a valuable tool in drug development and research.
Properties
Molecular Formula |
C27H38N2O4 |
---|---|
Molecular Weight |
460.6 g/mol |
IUPAC Name |
(2R)-2-(3,4-dimethoxyphenyl)-5-[2-(3,4-dimethoxyphenyl)ethyl-methylamino]-2-(1,1,1,3,3,3-hexadeuteriopropan-2-yl)pentanenitrile |
InChI |
InChI=1S/C27H38N2O4/c1-20(2)27(19-28,22-10-12-24(31-5)26(18-22)33-7)14-8-15-29(3)16-13-21-9-11-23(30-4)25(17-21)32-6/h9-12,17-18,20H,8,13-16H2,1-7H3/t27-/m1/s1/i1D3,2D3 |
InChI Key |
SGTNSNPWRIOYBX-SFGWWISVSA-N |
Isomeric SMILES |
[2H]C([2H])([2H])C([C@@](CCCN(C)CCC1=CC(=C(C=C1)OC)OC)(C#N)C2=CC(=C(C=C2)OC)OC)C([2H])([2H])[2H] |
Canonical SMILES |
CC(C)C(CCCN(C)CCC1=CC(=C(C=C1)OC)OC)(C#N)C2=CC(=C(C=C2)OC)OC |
Origin of Product |
United States |
Disclaimer and Information on In-Vitro Research Products
Please be aware that all articles and product information presented on BenchChem are intended solely for informational purposes. The products available for purchase on BenchChem are specifically designed for in-vitro studies, which are conducted outside of living organisms. In-vitro studies, derived from the Latin term "in glass," involve experiments performed in controlled laboratory settings using cells or tissues. It is important to note that these products are not categorized as medicines or drugs, and they have not received approval from the FDA for the prevention, treatment, or cure of any medical condition, ailment, or disease. We must emphasize that any form of bodily introduction of these products into humans or animals is strictly prohibited by law. It is essential to adhere to these guidelines to ensure compliance with legal and ethical standards in research and experimentation.