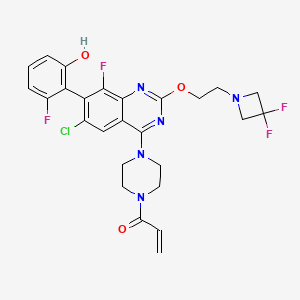
KRAS inhibitor-8
- Click on QUICK INQUIRY to receive a quote from our team of experts.
- With the quality product at a COMPETITIVE price, you can focus more on your research.
Overview
Description
KRAS inhibitor-8 is a small molecule designed to target the KRAS protein, which is a member of the RAS family of GTPases. KRAS mutations are implicated in a significant percentage of human cancers, including pancreatic, colorectal, and non-small cell lung cancers . The compound aims to inhibit the activity of KRAS, thereby disrupting the signaling pathways that lead to uncontrolled cell growth and proliferation.
Preparation Methods
Synthetic Routes and Reaction Conditions: The synthesis of KRAS inhibitor-8 involves multiple steps, including the formation of key intermediates and the final coupling reactions. The synthetic route typically starts with the preparation of a core scaffold, followed by functional group modifications to introduce the desired substituents . Common reagents used in these reactions include organic solvents, catalysts, and protective groups to ensure the selectivity and yield of the desired product.
Industrial Production Methods: Industrial production of this compound involves scaling up the laboratory synthesis to a larger scale while maintaining the purity and yield of the compound. This often requires optimization of reaction conditions, such as temperature, pressure, and solvent choice, as well as the use of continuous flow reactors to enhance efficiency and reproducibility .
Chemical Reactions Analysis
Types of Reactions: KRAS inhibitor-8 undergoes various chemical reactions, including oxidation, reduction, and substitution. These reactions are essential for modifying the compound’s structure to enhance its binding affinity and selectivity for the KRAS protein .
Common Reagents and Conditions: Common reagents used in these reactions include oxidizing agents like hydrogen peroxide, reducing agents like sodium borohydride, and nucleophiles for substitution reactions. The reaction conditions, such as temperature, pH, and solvent choice, are optimized to achieve the desired transformation with high yield and selectivity .
Major Products Formed: The major products formed from these reactions are typically intermediates that are further modified to produce the final this compound compound. These intermediates often contain functional groups that enhance the compound’s binding affinity and selectivity for the KRAS protein .
Scientific Research Applications
KRAS inhibitor-8 has a wide range of scientific research applications, particularly in the fields of chemistry, biology, medicine, and industry. In chemistry, it is used as a tool compound to study the structure and function of the KRAS protein and its interactions with other molecules . In biology, it is used to investigate the role of KRAS in cell signaling pathways and its impact on cell growth and proliferation . In medicine, this compound is being explored as a potential therapeutic agent for treating cancers driven by KRAS mutations . In industry, it is used in the development of new drugs and therapies targeting KRAS and related proteins .
Mechanism of Action
KRAS inhibitor-8 exerts its effects by binding to the KRAS protein and inhibiting its activity. The compound targets specific regions of the KRAS protein, such as the switch II pocket, to prevent the binding of GTP and GDP, which are essential for KRAS activation . By inhibiting KRAS activity, the compound disrupts downstream signaling pathways, such as the MAP kinase pathway, leading to reduced cell growth and proliferation .
Comparison with Similar Compounds
KRAS inhibitor-8 is unique compared to other KRAS inhibitors due to its specific binding affinity and selectivity for the KRAS protein. Similar compounds include sotorasib and adagrasib, which also target the KRAS protein but have different binding sites and mechanisms of action . This compound offers advantages in terms of its potency and ability to inhibit multiple KRAS mutants, making it a promising candidate for further development and clinical use .
List of Similar Compounds:- Sotorasib
- Adagrasib
- BI 2852
- MRTX849
- AMG 510
This compound stands out due to its unique binding properties and potential for broad-spectrum inhibition of KRAS mutants .
Properties
Molecular Formula |
C26H24ClF4N5O3 |
---|---|
Molecular Weight |
565.9 g/mol |
IUPAC Name |
1-[4-[6-chloro-2-[2-(3,3-difluoroazetidin-1-yl)ethoxy]-8-fluoro-7-(2-fluoro-6-hydroxyphenyl)quinazolin-4-yl]piperazin-1-yl]prop-2-en-1-one |
InChI |
InChI=1S/C26H24ClF4N5O3/c1-2-19(38)35-6-8-36(9-7-35)24-15-12-16(27)20(21-17(28)4-3-5-18(21)37)22(29)23(15)32-25(33-24)39-11-10-34-13-26(30,31)14-34/h2-5,12,37H,1,6-11,13-14H2 |
InChI Key |
ABVZCBJMBAVTME-UHFFFAOYSA-N |
Canonical SMILES |
C=CC(=O)N1CCN(CC1)C2=NC(=NC3=C(C(=C(C=C32)Cl)C4=C(C=CC=C4F)O)F)OCCN5CC(C5)(F)F |
Origin of Product |
United States |
Disclaimer and Information on In-Vitro Research Products
Please be aware that all articles and product information presented on BenchChem are intended solely for informational purposes. The products available for purchase on BenchChem are specifically designed for in-vitro studies, which are conducted outside of living organisms. In-vitro studies, derived from the Latin term "in glass," involve experiments performed in controlled laboratory settings using cells or tissues. It is important to note that these products are not categorized as medicines or drugs, and they have not received approval from the FDA for the prevention, treatment, or cure of any medical condition, ailment, or disease. We must emphasize that any form of bodily introduction of these products into humans or animals is strictly prohibited by law. It is essential to adhere to these guidelines to ensure compliance with legal and ethical standards in research and experimentation.