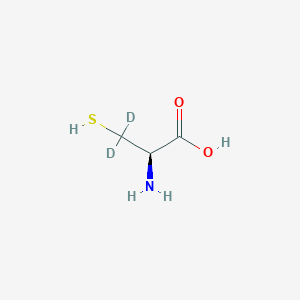
L-Cysteine-d2
- Click on QUICK INQUIRY to receive a quote from our team of experts.
- With the quality product at a COMPETITIVE price, you can focus more on your research.
Overview
Description
L-Cysteine-d2: is a deuterium-labeled derivative of L-Cysteine, an amino acid that plays a crucial role in various biological processes. The deuterium labeling involves the substitution of hydrogen atoms with deuterium, a stable isotope of hydrogen. This modification is often used in scientific research to study metabolic pathways and the pharmacokinetics of drugs. L-Cysteine itself is a conditionally essential amino acid, serving as a precursor for biologically active molecules such as hydrogen sulfide, glutathione, and taurine .
Preparation Methods
Synthetic Routes and Reaction Conditions: The synthesis of L-Cysteine-d2 typically involves the deuteration of L-Cysteine. One common method is the exchange of hydrogen atoms with deuterium using deuterated water (D2O) under specific conditions. This process can be catalyzed by various enzymes or chemical catalysts to ensure the efficient incorporation of deuterium atoms.
Industrial Production Methods: Industrial production of L-Cysteine, including its deuterated form, often involves the hydrolysis of proteins extracted from animal sources such as feathers or pig hair. This method, however, has environmental drawbacks due to the use of large amounts of hydrochloric acid and the generation of unpleasant odors and wastewater . To mitigate these issues, biotechnological approaches, including fermentation using genetically engineered microorganisms, have been developed. These methods offer a more sustainable and environmentally friendly alternative .
Chemical Reactions Analysis
Types of Reactions: L-Cysteine-d2 undergoes various chemical reactions, including:
Oxidation: this compound can be oxidized to form L-Cystine-d2, where two molecules of this compound are linked by a disulfide bond.
Reduction: The disulfide bond in L-Cystine-d2 can be reduced back to this compound.
Substitution: The thiol group in this compound can participate in substitution reactions, forming various derivatives.
Common Reagents and Conditions:
Oxidation: Hydrogen peroxide or molecular oxygen in the presence of a catalyst.
Reduction: Reducing agents such as dithiothreitol or β-mercaptoethanol.
Substitution: Alkylating agents like iodoacetamide for thiol group modifications.
Major Products:
Oxidation: L-Cystine-d2.
Reduction: this compound.
Substitution: Various alkylated derivatives depending on the substituent used
Scientific Research Applications
L-Cysteine-d2 has a wide range of applications in scientific research:
Chemistry: Used as a tracer in metabolic studies to understand the pathways and kinetics of sulfur-containing compounds.
Biology: Helps in studying the role of cysteine in cellular redox reactions and protein folding.
Medicine: Utilized in pharmacokinetic studies to track the metabolism and distribution of cysteine-containing drugs.
Industry: Employed in the production of food additives, cosmetics, and pharmaceuticals due to its antioxidant properties
Mechanism of Action
L-Cysteine-d2 exerts its effects primarily through its role in redox reactions and as a precursor for various biologically active molecules. It participates in the synthesis of glutathione, a critical antioxidant that protects cells from oxidative stress. The thiol group in this compound can undergo redox reactions, contributing to its antioxidant properties. Additionally, this compound is involved in the synthesis of hydrogen sulfide, which acts as a signaling molecule in various physiological processes .
Comparison with Similar Compounds
L-Cysteine: The non-deuterated form of L-Cysteine-d2, widely used in various biological and industrial applications.
L-Cystine: The oxidized dimer form of L-Cysteine, linked by a disulfide bond.
N-Acetylcysteine: A derivative of L-Cysteine used as a medication and dietary supplement.
Uniqueness: this compound is unique due to its deuterium labeling, which makes it particularly useful in tracing studies and pharmacokinetic analyses. The presence of deuterium atoms can alter the metabolic and pharmacokinetic profiles of the compound, providing valuable insights into its behavior in biological systems .
Properties
Molecular Formula |
C3H7NO2S |
---|---|
Molecular Weight |
123.17 g/mol |
IUPAC Name |
(2R)-2-amino-3,3-dideuterio-3-sulfanylpropanoic acid |
InChI |
InChI=1S/C3H7NO2S/c4-2(1-7)3(5)6/h2,7H,1,4H2,(H,5,6)/t2-/m0/s1/i1D2 |
InChI Key |
XUJNEKJLAYXESH-XFJCSJJYSA-N |
Isomeric SMILES |
[2H]C([2H])([C@@H](C(=O)O)N)S |
Canonical SMILES |
C(C(C(=O)O)N)S |
Origin of Product |
United States |
Disclaimer and Information on In-Vitro Research Products
Please be aware that all articles and product information presented on BenchChem are intended solely for informational purposes. The products available for purchase on BenchChem are specifically designed for in-vitro studies, which are conducted outside of living organisms. In-vitro studies, derived from the Latin term "in glass," involve experiments performed in controlled laboratory settings using cells or tissues. It is important to note that these products are not categorized as medicines or drugs, and they have not received approval from the FDA for the prevention, treatment, or cure of any medical condition, ailment, or disease. We must emphasize that any form of bodily introduction of these products into humans or animals is strictly prohibited by law. It is essential to adhere to these guidelines to ensure compliance with legal and ethical standards in research and experimentation.