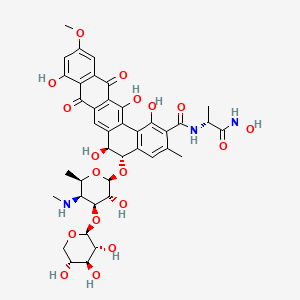
Antifungal agent 26
- Click on QUICK INQUIRY to receive a quote from our team of experts.
- With the quality product at a COMPETITIVE price, you can focus more on your research.
Overview
Description
Antifungal agent 26 is a synthetic compound designed to combat fungal infections. It belongs to a class of antifungal agents that target specific components of fungal cells, thereby inhibiting their growth and proliferation. This compound is particularly effective against a wide range of pathogenic fungi, making it a valuable tool in both clinical and agricultural settings.
Preparation Methods
Synthetic Routes and Reaction Conditions
The synthesis of Antifungal agent 26 typically involves a multi-step process. The initial step often includes the formation of a core structure through a series of condensation reactions. This is followed by the introduction of functional groups that enhance the antifungal activity of the compound. Common reagents used in these reactions include various organic solvents, catalysts, and protective groups to ensure the stability of intermediate products.
Industrial Production Methods
In an industrial setting, the production of this compound is scaled up using batch or continuous flow reactors. The reaction conditions are optimized to maximize yield and purity while minimizing the formation of by-products. Quality control measures, such as high-performance liquid chromatography and mass spectrometry, are employed to ensure the consistency and efficacy of the final product.
Chemical Reactions Analysis
Types of Reactions
Antifungal agent 26 undergoes several types of chemical reactions, including:
Oxidation: This reaction involves the addition of oxygen or the removal of hydrogen, often facilitated by oxidizing agents like hydrogen peroxide or potassium permanganate.
Reduction: The compound can also undergo reduction reactions, where hydrogen is added or oxygen is removed, typically using reducing agents such as sodium borohydride.
Substitution: In these reactions, one functional group in the molecule is replaced by another. Common reagents include halogens and nucleophiles.
Common Reagents and Conditions
Oxidizing Agents: Hydrogen peroxide, potassium permanganate.
Reducing Agents: Sodium borohydride, lithium aluminum hydride.
Substitution Reagents: Halogens, nucleophiles like amines and thiols.
Major Products
The major products formed from these reactions depend on the specific conditions and reagents used. For example, oxidation may yield hydroxylated derivatives, while reduction could produce deoxygenated compounds. Substitution reactions typically result in the formation of new functionalized derivatives of this compound.
Scientific Research Applications
Antifungal agent 26 has a broad range of applications in scientific research:
Chemistry: It is used as a model compound to study the mechanisms of antifungal activity and to develop new synthetic routes for antifungal agents.
Biology: Researchers use it to investigate the cellular processes affected by antifungal agents and to identify potential resistance mechanisms in fungi.
Medicine: Clinically, it is employed in the treatment of fungal infections, particularly those resistant to conventional antifungal drugs.
Industry: In agriculture, this compound is used to protect crops from fungal pathogens, thereby improving yield and quality.
Mechanism of Action
The primary mechanism of action of Antifungal agent 26 involves the inhibition of ergosterol synthesis, a crucial component of fungal cell membranes. By blocking the enzyme lanosterol 14α-demethylase, the compound disrupts the integrity of the cell membrane, leading to increased permeability and cell death. This mechanism is similar to that of other azole antifungals but with enhanced efficacy and reduced resistance.
Comparison with Similar Compounds
Similar Compounds
- Fluconazole
- Itraconazole
- Ketoconazole
- Voriconazole
Uniqueness
Compared to these similar compounds, Antifungal agent 26 exhibits a broader spectrum of activity and a lower propensity for resistance development. Its unique chemical structure allows for more effective binding to the target enzyme, resulting in higher antifungal potency. Additionally, it has a better safety profile, with fewer side effects observed in clinical trials.
Properties
Molecular Formula |
C40H45N3O18 |
---|---|
Molecular Weight |
855.8 g/mol |
IUPAC Name |
(5S,6S)-1,6,9,14-tetrahydroxy-N-[(2R)-1-(hydroxyamino)-1-oxopropan-2-yl]-5-[(2S,3R,4S,5S,6R)-3-hydroxy-6-methyl-5-(methylamino)-4-[(2S,3R,4S,5R)-3,4,5-trihydroxyoxan-2-yl]oxyoxan-2-yl]oxy-11-methoxy-3-methyl-8,13-dioxo-5,6-dihydrobenzo[a]tetracene-2-carboxamide |
InChI |
InChI=1S/C40H45N3O18/c1-11-6-18-24(31(50)21(11)38(55)42-12(2)37(54)43-56)23-16(9-17-25(32(23)51)28(47)15-7-14(57-5)8-19(44)22(15)27(17)46)29(48)35(18)60-40-34(53)36(26(41-4)13(3)59-40)61-39-33(52)30(49)20(45)10-58-39/h6-9,12-13,20,26,29-30,33-36,39-41,44-45,48-53,56H,10H2,1-5H3,(H,42,55)(H,43,54)/t12-,13-,20-,26+,29+,30+,33-,34-,35+,36+,39+,40+/m1/s1 |
InChI Key |
KEIDQQKYKNPXMT-NJGWPHBESA-N |
Isomeric SMILES |
C[C@@H]1[C@@H]([C@@H]([C@H]([C@@H](O1)O[C@@H]2[C@H](C3=CC4=C(C(=C3C5=C2C=C(C(=C5O)C(=O)N[C@H](C)C(=O)NO)C)O)C(=O)C6=C(C4=O)C(=CC(=C6)OC)O)O)O)O[C@H]7[C@@H]([C@H]([C@@H](CO7)O)O)O)NC |
Canonical SMILES |
CC1C(C(C(C(O1)OC2C(C3=CC4=C(C(=C3C5=C2C=C(C(=C5O)C(=O)NC(C)C(=O)NO)C)O)C(=O)C6=C(C4=O)C(=CC(=C6)OC)O)O)O)OC7C(C(C(CO7)O)O)O)NC |
Origin of Product |
United States |
Disclaimer and Information on In-Vitro Research Products
Please be aware that all articles and product information presented on BenchChem are intended solely for informational purposes. The products available for purchase on BenchChem are specifically designed for in-vitro studies, which are conducted outside of living organisms. In-vitro studies, derived from the Latin term "in glass," involve experiments performed in controlled laboratory settings using cells or tissues. It is important to note that these products are not categorized as medicines or drugs, and they have not received approval from the FDA for the prevention, treatment, or cure of any medical condition, ailment, or disease. We must emphasize that any form of bodily introduction of these products into humans or animals is strictly prohibited by law. It is essential to adhere to these guidelines to ensure compliance with legal and ethical standards in research and experimentation.