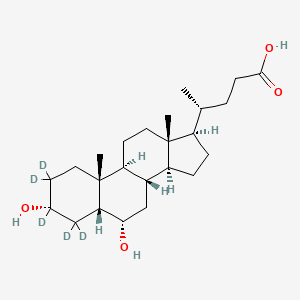
Hyodeoxycholic Acid-d5
- Click on QUICK INQUIRY to receive a quote from our team of experts.
- With the quality product at a COMPETITIVE price, you can focus more on your research.
Overview
Description
Hyodeoxycholic Acid-d5 (HDCA-d5) is a deuterium-labeled isotopologue of hyodeoxycholic acid (HDCA), a secondary bile acid naturally found in mammals. HDCA-d5 is synthesized by replacing five hydrogen atoms with deuterium at specific positions, enhancing its utility as an internal standard in mass spectrometry-based metabolic studies . Its molecular formula is C₂₄H₃₅D₅O₄, with a molecular weight of 397.62 g/mol (vs. 392.58 g/mol for non-deuterated HDCA) . Structurally, HDCA features hydroxyl groups at positions 3α and 6α on the steroid nucleus, distinguishing it from other bile acids like cholic acid (3α,7α,12α-trihydroxy) and chenodeoxycholic acid (3α,7α-dihydroxy) .
HDCA-d5 is pivotal in quantifying endogenous bile acids in biological samples due to its isotopic stability and minimal interference with analytical signals .
Preparation Methods
Synthetic Routes and Reaction Conditions
Hyodeoxycholic Acid-d5 is synthesized by introducing deuterium atoms into the Hyodeoxycholic Acid molecule. The process typically involves the hydrogen-deuterium exchange reaction, where hydrogen atoms in the Hyodeoxycholic Acid are replaced with deuterium. This can be achieved using deuterated reagents under specific reaction conditions, such as elevated temperatures and the presence of a catalyst.
Industrial Production Methods
Industrial production of this compound involves large-scale hydrogen-deuterium exchange reactions. The process is optimized to ensure high yield and purity of the deuterium-labeled compound. The production is carried out in specialized reactors designed to handle deuterated reagents and maintain the integrity of the deuterium labeling.
Chemical Reactions Analysis
Chemical Reactions of Hyodeoxycholic Acid-d5
HDCA-d5 participates in several chemical reactions typical of bile acids:
-
Esterification Reactions: These reactions can modify the compound's functional groups to create derivatives that may have enhanced solubility or bioactivity.
-
Conjugation: HDCA-d5 can undergo conjugation with amino acids (such as glycine and taurine) or sulfate groups, which is crucial for its biological function and solubility .
The reaction conditions (e.g., temperature, pH) must be optimized to ensure efficient conversion while minimizing side reactions.
Mechanisms of Action
The mechanism by which this compound exerts its effects involves several pathways:
-
Activation of GPCRs: As a bile acid, α-Hyodeoxycholic Acid-d5 functions by activating specific G protein-coupled receptors (GPCRs), such as TGR5 (GPCR19). This activation plays a role in regulating glucose metabolism and energy expenditure.
-
Influence on Lipid Metabolism: Research indicates that this compound may stimulate hepatic pathways that lead to altered lipid profiles in animal models.
Comparison with Other Bile Acids
Compound Name | Structural Features | Unique Aspects |
---|---|---|
Deoxycholic Acid | Non-deuterated form; lacks neurotrophic effects | More commonly studied for lipid digestion |
Chenodeoxycholic Acid | Has different hydroxyl group positioning | Stronger influence on cholesterol metabolism |
Ursodeoxycholic Acid | Known for protective effects on liver function | Used clinically for liver diseases |
This compound | Stable isotope labeling with five deuterium atoms, enhancing stability for tracing and metabolic studies. Hydrophilic properties due to hydroxyl groups. | Utility in tracing studies and pharmacological research while maintaining similar biological activities as other bile acids. Useful as an internal standard in mass spectrometry. |
HDCA-d5 stands out due to its stable isotope labeling, which enhances its utility in tracing studies and pharmacological research while maintaining similar biological activities as other bile acids.
Scientific Research Applications
Therapeutic Potential in Liver Diseases
Recent research led by Hong Kong Baptist University has demonstrated that hyodeoxycholic acid can significantly reduce fat accumulation and inflammation in the liver, showcasing its potential as a treatment for non-alcoholic fatty liver disease (NAFLD). The study found that HDCA-d5 alters the gut microbiome, particularly increasing the population of Parabacteroides distasonis, which plays a crucial role in fatty acid metabolism and hepatic bile acid synthesis pathways. This alteration suggests that HDCA-d5 could be instrumental in managing liver diseases through modulation of the gut-liver axis .
Role in Diabetes Management
Hyodeoxycholic acid has also shown promise in the management of type 2 diabetes. A study investigated the use of HDCA-d5 to modify metformin liposomes, enhancing their efficacy and bioavailability. The modified liposomes were shown to improve glucose homeostasis by upregulating glucagon-like peptide-1 (GLP-1) secretion and subsequently increasing insulin production. This positions HDCA-d5 as a potential adjunct therapy for diabetes, addressing common issues associated with metformin such as gastrointestinal intolerance .
Drug Delivery Systems
The application of hyodeoxycholic acid in drug delivery systems is another significant area of research. By modifying liposomes with HDCA-d5, researchers have been able to create more effective delivery vehicles for anti-diabetic medications. The thin-film dispersion method was employed to prepare various liposome formulations containing different ratios of HDCA-d5 and metformin, which were then characterized for their drug release profiles and biological activity .
Summary of Research Findings
The following table summarizes key findings from recent studies on the applications of hyodeoxycholic acid-d5:
Case Studies
- Non-Alcoholic Fatty Liver Disease : In a controlled study, mice treated with HDCA exhibited a significant reduction in liver fat content compared to control groups. The intervention also led to improved metabolic markers associated with liver function.
- Type 2 Diabetes : Clinical trials involving HDCA-modified metformin liposomes showed enhanced glycemic control in diabetic models, indicating a promising direction for future diabetes therapies.
Mechanism of Action
Hyodeoxycholic Acid-d5 exerts its effects by acting as an agonist for the TGR5 receptor. This receptor is involved in various physiological processes, including the regulation of glucose homeostasis and lipid metabolism. The activation of TGR5 by this compound leads to the modulation of signaling pathways that influence these metabolic processes .
Comparison with Similar Compounds
Structural and Functional Differences
Table 1: Structural Comparison of HDCA-d5 and Key Bile Acids
Key Observations :
- Hydroxylation Pattern: HDCA and HDCA-d5 share 3α,6α-dihydroxy groups, whereas chenodeoxycholic acid (CDCA) and ursodeoxycholic acid (UDCA) differ in hydroxyl positions (7α vs. 7β), impacting receptor specificity (e.g., FXR vs. TGR5 activation) .
- Deuterium Labeling: HDCA-d5’s isotopic labeling enables precise quantification in complex matrices, a feature absent in non-deuterated analogs .
Metabolic and Therapeutic Profiles
Key Findings :
- HDCA vs. HDCA-d5: Non-deuterated HDCA reduces plasma cholesterol by up to 62% in LDLR-knockout mice and suppresses aortic root lesions by 80%, while HDCA-d5 is exclusively used for analytical purposes .
- Gallstone Prevention : HDCA prevents gallstones in hamsters by reducing cholesterol absorption, unlike UDCA and hyocholic acid .
- Receptor Interactions : HDCA activates TGR5 to enhance GLP-1 secretion, whereas CDCA primarily targets FXR, which regulates bile acid homeostasis .
Analytical Utility :
Biological Activity
Hyodeoxycholic Acid-d5 (HDCA-d5) is a deuterated derivative of hyodeoxycholic acid, a secondary bile acid that plays a significant role in various biological processes. This article delves into the biological activity of HDCA-d5, focusing on its effects on liver health, metabolic regulation, and potential therapeutic applications.
Overview of Hyodeoxycholic Acid
Hyodeoxycholic acid (HDCA) is primarily produced in the intestine through the metabolism of primary bile acids by gut microbiota. It exhibits unique properties that differentiate it from other bile acids, such as deoxycholic acid (DCA) and ursodeoxycholic acid (UDCA). HDCA is known for its hydrophilic nature and its ability to modulate lipid metabolism and gut microbiota composition, making it a compound of interest in metabolic diseases like non-alcoholic fatty liver disease (NAFLD) .
1. Modulation of Gut-Liver Axis
Research indicates that HDCA influences the gut-liver axis by altering the composition of gut microbiota. In a study involving mouse models of NAFLD, HDCA treatment was associated with increased levels of beneficial bacteria such as Parabacteroides distasonis, which enhances lipid catabolism through the activation of hepatic peroxisome proliferator-activated receptor alpha (PPARα) signaling . This mechanism suggests that HDCA can alleviate liver inflammation and fat accumulation by reshaping gut microbiota.
2. Regulation of Bile Acid Synthesis
HDCA has been shown to inhibit the farnesoid X receptor (FXR), leading to an upregulation of hepatic CYP7B1, an enzyme involved in bile acid synthesis. This dual action not only reduces bile acid toxicity but also promotes healthier bile acid profiles, which are crucial for maintaining metabolic homeostasis .
1. Non-Alcoholic Fatty Liver Disease (NAFLD)
A pivotal study highlighted HDCA's therapeutic potential in treating NAFLD. The research demonstrated that individuals with NAFLD had lower levels of HDCA compared to healthy controls. Supplementation with HDCA improved liver health by reducing fat accumulation and inflammation . A clinical trial is underway to evaluate its efficacy in patients with fatty liver disease and type 2 diabetes .
2. Cholesterol Metabolism and Atherosclerosis
HDCA has been identified as a candidate for anti-atherosclerotic therapy due to its ability to improve high-density lipoprotein (HDL) function and inhibit atherosclerotic lesion formation in animal models. It reduces intestinal cholesterol absorption significantly, thereby lowering plasma cholesterol levels . This property positions HDCA as a potential agent for managing cardiovascular diseases linked to dyslipidemia.
Case Studies
Case Study 1: Effects on NAFLD
- Population: 178 patients with NAFLD vs. 73 healthy controls
- Findings: Patients with NAFLD exhibited significantly lower levels of HDCA, suggesting a correlation between HDCA levels and disease severity.
- Outcome: HDCA supplementation resulted in improved liver function tests and reduced hepatic fat content.
Case Study 2: Impact on Atherosclerosis
- Animal Model: LDLR-knockout mice
- Intervention: HDCA supplementation
- Results:
- Atherosclerotic lesions decreased by up to 94% in treated groups.
- Significant reduction in VLDL/IDL/LDL cholesterol levels.
Data Summary Table
Biological Activity | Effect | Mechanism |
---|---|---|
Alleviation of NAFLD | Reduced liver fat accumulation | Modulation of gut microbiota and FXR inhibition |
Anti-atherosclerotic | Decreased lesion size | Inhibition of intestinal cholesterol absorption |
Gallstone prevention | Prevented cholesterol gallstones | Inhibition of HMG-CoA reductase activity |
Q & A
Basic Research Questions
Q. What are the primary research applications of Hyodeoxycholic Acid-d5 in metabolic studies?
this compound (HDCA-d5) is primarily used as a stable isotope-labeled tracer to investigate bile acid metabolism, lipid homeostasis, and receptor-mediated signaling pathways. Its deuterated structure enables precise tracking in:
- LC-MS/MS quantification : Acts as an internal standard for quantifying endogenous HDCA levels in biological matrices (e.g., plasma, liver tissue) .
- Metabolic flux analysis : Tracks cholesterol metabolism and bile acid synthesis kinetics in in vitro hepatocyte models or in vivo systems .
- Gut-liver axis studies : Examines microbial modulation of bile acid profiles using isotope dilution mass spectrometry .
Q. What analytical techniques are recommended for quantifying this compound in biological samples?
- Liquid Chromatography-Tandem Mass Spectrometry (LC-MS/MS) :
- Sample preparation : Use protein precipitation with deuterated internal standards (e.g., HDCA-d5) to minimize matrix effects.
- Chromatographic conditions : Reverse-phase C18 columns with mobile phases of methanol/water + 0.1% formic acid .
- Detection : Multiple Reaction Monitoring (MRM) transitions specific to HDCA-d5 (e.g., m/z 397 → 379 for quantification) .
- Nuclear Magnetic Resonance (NMR) : Validates structural integrity and isotopic purity in synthesized HDCA-d5 batches .
Q. How can researchers optimize the synthesis of this compound for high isotopic purity?
Deuterium incorporation into HDCA is achieved via:
- Biocatalytic methods : Lipase-mediated regioselective acetylation/esterification under mild conditions (e.g., 40°C, pH 7.5) to preserve deuterium labels .
- Key parameters :
- Enzyme selection : Candida antarctica lipase B achieves >90% yield with minimal side reactions.
- Solvent optimization : Use tert-amyl alcohol to enhance solubility and reaction efficiency .
- Isotopic purity validation : Confirm via high-resolution MS and NMR .
Advanced Research Questions
Q. How does this compound interact with Farnesoid X Receptor (FXR) in mechanistic studies?
HDCA-d5 is used to dissect FXR activation dynamics:
- Competitive binding assays : Co-incubate HDCA-d5 with radiolabeled FXR ligands (e.g., ^3H-CDCA) to measure displacement efficacy .
- Transcriptomic profiling : Combine HDCA-d5 treatment with RNA-seq to identify FXR-regulated genes (e.g., SHP, BSEP) in hepatoma cell lines .
- Dose-response studies : Establish EC₅₀ values for HDCA-d5 using luciferase reporter assays in FXR-transfected HEK293 cells .
Q. What experimental strategies resolve contradictory data on HDCA-d5’s role in cholesterol metabolism?
Contradictions often arise from model-specific variables:
- Species differences : Compare HDCA-d5 effects in human primary hepatocytes vs. rodent models .
- Dosage optimization : Titrate HDCA-d5 concentrations (0.1–100 µM) to assess dose-dependent modulation of HMG-CoA reductase activity .
- Co-factor analysis : Evaluate interactions with lipid transporters (e.g., NPC1L1) using siRNA knockdown + HDCA-d5 tracing .
Q. How is HDCA-d5 applied in metabolic flux analysis of bile acid enterohepatic circulation?
- Isotope tracing : Administer ^13C/HDCA-d5 orally to mice and monitor isotopic enrichment in portal blood and fecal samples via LC-MS .
- Kinetic modeling : Calculate turnover rates using compartmental models (e.g., SAAM II software) to quantify hepatic uptake and intestinal reabsorption .
- Microbiota-depleted models : Use germ-free mice to isolate host vs. microbial contributions to HDCA-d5 metabolism .
Q. What challenges exist in maintaining HDCA-d5 stability during long-term experiments?
- Storage conditions : Store lyophilized HDCA-d5 at -80°C under argon to prevent deuterium exchange .
- Solvent selection : Avoid aqueous buffers at high pH; use methanol/ethanol for stock solutions to minimize degradation .
- Stability monitoring : Conduct periodic LC-MS checks for deuterium loss or oxidation byproducts .
Q. Methodological Considerations
Q. How to validate HDCA-d5’s role in gut microbiota interactions?
- Gnotobiotic models : Colonize germ-free mice with defined microbial consortia and track HDCA-d5 metabolism via metagenomics/metabolomics .
- Fecal fermentation assays : Incubate HDCA-d5 with human fecal slurries under anaerobic conditions to identify microbial transformation products (e.g., dehydroxylated derivatives) .
Q. What statistical approaches are recommended for analyzing HDCA-d5 tracer data?
Properties
Molecular Formula |
C24H40O4 |
---|---|
Molecular Weight |
397.6 g/mol |
IUPAC Name |
(4R)-4-[(3R,5R,6S,8S,9S,10R,13R,14S,17R)-2,2,3,4,4-pentadeuterio-3,6-dihydroxy-10,13-dimethyl-1,5,6,7,8,9,11,12,14,15,16,17-dodecahydrocyclopenta[a]phenanthren-17-yl]pentanoic acid |
InChI |
InChI=1S/C24H40O4/c1-14(4-7-22(27)28)17-5-6-18-16-13-21(26)20-12-15(25)8-10-24(20,3)19(16)9-11-23(17,18)2/h14-21,25-26H,4-13H2,1-3H3,(H,27,28)/t14-,15-,16+,17-,18+,19+,20+,21+,23-,24-/m1/s1/i8D2,12D2,15D |
InChI Key |
DGABKXLVXPYZII-QJMAJIAASA-N |
Isomeric SMILES |
[2H][C@]1(C(C[C@@]2([C@H]3CC[C@]4([C@H]([C@@H]3C[C@@H]([C@@H]2C1([2H])[2H])O)CC[C@@H]4[C@H](C)CCC(=O)O)C)C)([2H])[2H])O |
Canonical SMILES |
CC(CCC(=O)O)C1CCC2C1(CCC3C2CC(C4C3(CCC(C4)O)C)O)C |
Origin of Product |
United States |
Disclaimer and Information on In-Vitro Research Products
Please be aware that all articles and product information presented on BenchChem are intended solely for informational purposes. The products available for purchase on BenchChem are specifically designed for in-vitro studies, which are conducted outside of living organisms. In-vitro studies, derived from the Latin term "in glass," involve experiments performed in controlled laboratory settings using cells or tissues. It is important to note that these products are not categorized as medicines or drugs, and they have not received approval from the FDA for the prevention, treatment, or cure of any medical condition, ailment, or disease. We must emphasize that any form of bodily introduction of these products into humans or animals is strictly prohibited by law. It is essential to adhere to these guidelines to ensure compliance with legal and ethical standards in research and experimentation.