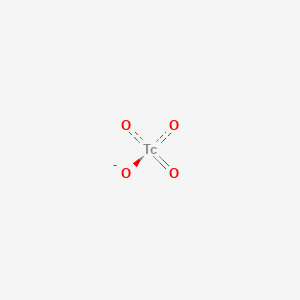
Pertechnetate
- Click on QUICK INQUIRY to receive a quote from our team of experts.
- With the quality product at a COMPETITIVE price, you can focus more on your research.
Overview
Description
Pertechnetate is an oxyanion with the chemical formula TcO₄⁻. It is a water-soluble source of isotopes of the radioactive element technetium. This compound is often used in nuclear medicine, particularly for carrying the isotope technetium-99m, which is widely used in various nuclear scanning procedures .
Scientific Research Applications
Pertechnetate has a wide range of applications in scientific research, including:
Preparation Methods
Synthetic Routes and Reaction Conditions: Pertechnetate is produced by oxidizing technetium with nitric acid or hydrogen peroxide. The this compound anion is similar to the permanganate anion but is a weaker oxidizing agent .
Industrial Production Methods: Technetium-99m is conveniently available in high radionuclidic purity from molybdenum-99, which decays to technetium-99m. Molybdenum-99 can be produced in a nuclear reactor via irradiation of molybdenum-98 or naturally occurring molybdenum with thermal neutrons. Currently, molybdenum-99 is recovered as a product of the nuclear fission reaction of uranium-235, separated from other fission products via a multistep process, and loaded onto a column of alumina that forms the core of a molybdenum-99/technetium-99m radioisotope generator .
Chemical Reactions Analysis
Types of Reactions: Pertechnetate undergoes various types of reactions, including reduction and complexation. It is a weaker oxidizing agent compared to permanganate but can be reduced to lower oxidation states of technetium, such as technetium (VI), technetium (V), and technetium (IV) .
Common Reagents and Conditions:
Reduction: this compound can be reduced using reducing agents like hydrogen peroxide or nitric acid.
Complexation: It can form complex salts with metals like actinides, barium, scandium, yttrium, or zirconium.
Major Products:
Reduction Products: Depending on the reducing agent, this compound can be converted to derivatives containing technetium (VI), technetium (V), and technetium (IV).
Complex Salts: Complex salts with metals like actinides, barium, scandium, yttrium, or zirconium.
Mechanism of Action
The pertechnetate ion distributes in the body similarly to the iodide ion but is not organified when trapped in the thyroid gland. It concentrates in the thyroid gland, salivary glands, gastric mucosa, and choroid plexus. The uptake of the this compound anion in the thyroid parenchyma is mediated by the sodium-iodide symporter, a transmembrane glycoprotein .
Comparison with Similar Compounds
Permanganate (MnO₄⁻): Strong oxidizing agent.
Perrhenate (ReO₄⁻): Similar to pertechnetate but with lower oxidation power.
Perchlorate (ClO₄⁻): Strong oxidizing agent.
Perbromate (BrO₄⁻): Strong oxidizing agent.
Periodate (IO₄⁻): Strong oxidizing agent.
This compound’s unique properties, such as its stability in alkaline solutions and its ability to form complex salts with various metals, make it a valuable compound in various scientific and industrial applications.
Properties
Molecular Formula |
O4Tc- |
---|---|
Molecular Weight |
160.904 g/mol |
IUPAC Name |
oxido(trioxo)technetium |
InChI |
InChI=1S/4O.Tc/q;;;-1; |
InChI Key |
FXUHHSACBOWLSP-UHFFFAOYSA-N |
SMILES |
[O-][Tc](=O)(=O)=O |
Canonical SMILES |
[O-][Tc](=O)(=O)=O |
Synonyms |
99m-Pertechnetate, Tc 99mTcO4 99Tc Pertechnetate 99Tc-Pertechnetate 99TcmO4 Pertechnetate Pertechnetate Sodium Pertechnetate, Technetium Sodium Pertechnetate Tc 99m Sodium, Pertechnetate Tc 99m Pertechnetate Tc 99m-Pertechnetate Technetium 99mTc pertechnetate Technetium Pertechnetate Technetium Tc 99m O(4) Technetium Tc 99m Pertechnetate Technetium-99mTc-pertechnetate |
Origin of Product |
United States |
Disclaimer and Information on In-Vitro Research Products
Please be aware that all articles and product information presented on BenchChem are intended solely for informational purposes. The products available for purchase on BenchChem are specifically designed for in-vitro studies, which are conducted outside of living organisms. In-vitro studies, derived from the Latin term "in glass," involve experiments performed in controlled laboratory settings using cells or tissues. It is important to note that these products are not categorized as medicines or drugs, and they have not received approval from the FDA for the prevention, treatment, or cure of any medical condition, ailment, or disease. We must emphasize that any form of bodily introduction of these products into humans or animals is strictly prohibited by law. It is essential to adhere to these guidelines to ensure compliance with legal and ethical standards in research and experimentation.