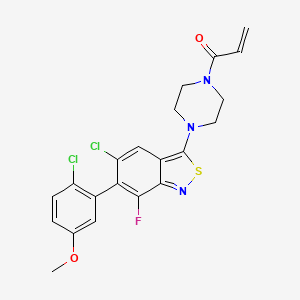
KRAS inhibitor-17
- Click on QUICK INQUIRY to receive a quote from our team of experts.
- With the quality product at a COMPETITIVE price, you can focus more on your research.
Overview
Description
KRAS inhibitor-17 is a small molecule compound designed to inhibit the activity of the KRAS protein, which is a small GTPase involved in cell signaling pathways that regulate cell growth and proliferation. KRAS mutations are among the most common oncogenic drivers in human cancers, particularly in non-small cell lung cancer, colorectal cancer, and pancreatic ductal adenocarcinoma . This compound specifically targets mutant forms of KRAS, making it a promising candidate for cancer therapy.
Preparation Methods
Synthetic Routes and Reaction Conditions
The synthesis of KRAS inhibitor-17 involves multiple steps, including the formation of key intermediates and the final coupling reactions. One common synthetic route includes the following steps:
Formation of the Core Structure: The core structure of this compound is synthesized through a series of condensation and cyclization reactions. These reactions typically involve the use of reagents such as aldehydes, amines, and carboxylic acids under controlled conditions.
Functional Group Modifications: The core structure is then modified to introduce specific functional groups that enhance the binding affinity and selectivity of the compound. This may involve reactions such as halogenation, alkylation, and acylation.
Final Coupling Reactions: The final step involves coupling the modified core structure with other molecular fragments to complete the synthesis of this compound.
Industrial Production Methods
Industrial production of this compound follows similar synthetic routes but is optimized for large-scale manufacturing. This includes the use of continuous flow reactors, automated synthesis platforms, and advanced purification techniques to ensure high yield and purity of the final product .
Chemical Reactions Analysis
Types of Reactions
KRAS inhibitor-17 undergoes various chemical reactions, including:
Oxidation: The compound can be oxidized under specific conditions to form oxidized derivatives. Common oxidizing agents include hydrogen peroxide and potassium permanganate.
Reduction: Reduction reactions can be performed using reducing agents such as sodium borohydride or lithium aluminum hydride to obtain reduced forms of the compound.
Substitution: Substitution reactions involve the replacement of specific functional groups with other groups.
Common Reagents and Conditions
Oxidation: Hydrogen peroxide, potassium permanganate, and other oxidizing agents under acidic or basic conditions.
Reduction: Sodium borohydride, lithium aluminum hydride, and other reducing agents under anhydrous conditions.
Substitution: Halogens, alkyl halides, and nucleophiles under various solvent conditions.
Major Products Formed
The major products formed from these reactions include oxidized, reduced, and substituted derivatives of this compound. These derivatives can be further analyzed for their biological activity and potential therapeutic applications .
Scientific Research Applications
KRAS inhibitor-17 has a wide range of scientific research applications, including:
Chemistry: The compound is used as a tool for studying the chemical properties and reactivity of KRAS inhibitors. It is also used in the development of new synthetic methodologies for targeting KRAS.
Biology: In biological research, this compound is used to study the role of KRAS in cell signaling pathways and its impact on cell growth and proliferation. It is also used in the development of new assays for detecting KRAS activity.
Medicine: this compound is a promising candidate for cancer therapy, particularly for cancers driven by KRAS mutations. It is used in preclinical and clinical studies to evaluate its efficacy and safety as a therapeutic agent.
Industry: The compound is used in the pharmaceutical industry for the development of new cancer therapies.
Mechanism of Action
KRAS inhibitor-17 exerts its effects by binding to the KRAS protein and inhibiting its activity. The compound specifically targets the switch regions of KRAS, which are critical for its interaction with downstream signaling molecules. By binding to these regions, this compound prevents the activation of KRAS and its downstream signaling pathways, leading to the inhibition of cell growth and proliferation .
Comparison with Similar Compounds
KRAS inhibitor-17 is unique in its ability to specifically target mutant forms of KRAS, making it more selective and effective compared to other KRAS inhibitors. Similar compounds include:
Sotorasib: A KRAS G12C inhibitor that has been approved for the treatment of non-small cell lung cancer.
Adagrasib: Another KRAS G12C inhibitor that is currently being investigated in clinical trials.
BI 1701963: A pan-KRAS inhibitor that targets multiple KRAS mutations and is in clinical development
These compounds share similar mechanisms of action but differ in their selectivity, potency, and clinical applications. This compound stands out due to its unique binding properties and potential for broader therapeutic applications .
Properties
Molecular Formula |
C21H18Cl2FN3O2S |
---|---|
Molecular Weight |
466.4 g/mol |
IUPAC Name |
1-[4-[5-chloro-6-(2-chloro-5-methoxyphenyl)-7-fluoro-2,1-benzothiazol-3-yl]piperazin-1-yl]prop-2-en-1-one |
InChI |
InChI=1S/C21H18Cl2FN3O2S/c1-3-17(28)26-6-8-27(9-7-26)21-14-11-16(23)18(19(24)20(14)25-30-21)13-10-12(29-2)4-5-15(13)22/h3-5,10-11H,1,6-9H2,2H3 |
InChI Key |
UPTMOORQEKJHRA-UHFFFAOYSA-N |
Canonical SMILES |
COC1=CC(=C(C=C1)Cl)C2=C(C3=NSC(=C3C=C2Cl)N4CCN(CC4)C(=O)C=C)F |
Origin of Product |
United States |
Disclaimer and Information on In-Vitro Research Products
Please be aware that all articles and product information presented on BenchChem are intended solely for informational purposes. The products available for purchase on BenchChem are specifically designed for in-vitro studies, which are conducted outside of living organisms. In-vitro studies, derived from the Latin term "in glass," involve experiments performed in controlled laboratory settings using cells or tissues. It is important to note that these products are not categorized as medicines or drugs, and they have not received approval from the FDA for the prevention, treatment, or cure of any medical condition, ailment, or disease. We must emphasize that any form of bodily introduction of these products into humans or animals is strictly prohibited by law. It is essential to adhere to these guidelines to ensure compliance with legal and ethical standards in research and experimentation.