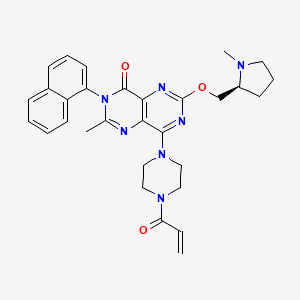
KRAS G12C inhibitor 33
- Click on QUICK INQUIRY to receive a quote from our team of experts.
- With the quality product at a COMPETITIVE price, you can focus more on your research.
Overview
Description
KRAS G12C inhibitor 33 is a small molecule designed to target the KRAS G12C mutation, a common oncogenic driver in various cancers, particularly non-small cell lung cancer (NSCLC), colorectal cancer, and pancreatic ductal adenocarcinoma. This mutation involves the substitution of glycine with cysteine at position 12 of the KRAS protein, leading to uncontrolled cell proliferation and tumor growth .
Preparation Methods
Synthetic Routes and Reaction Conditions
The synthesis of KRAS G12C inhibitor 33 involves multiple steps, including the formation of key intermediates and final coupling reactions. The synthetic route typically starts with the preparation of a core scaffold, followed by functional group modifications to introduce the desired pharmacophores. Common reagents used in these reactions include organometallic reagents, protecting groups, and coupling agents .
Industrial Production Methods
Industrial production of this compound involves scaling up the laboratory synthesis to a larger scale while ensuring the purity and yield of the final product. This process often requires optimization of reaction conditions, purification techniques, and quality control measures to meet regulatory standards .
Chemical Reactions Analysis
Types of Reactions
KRAS G12C inhibitor 33 undergoes various chemical reactions, including:
Oxidation: Introduction of oxygen atoms to form oxides or hydroxides.
Reduction: Removal of oxygen or addition of hydrogen to form reduced products.
Substitution: Replacement of one functional group with another, often using nucleophilic or electrophilic reagents
Common Reagents and Conditions
Common reagents used in these reactions include oxidizing agents like hydrogen peroxide, reducing agents like sodium borohydride, and nucleophiles like amines or thiols. Reaction conditions often involve controlled temperatures, pH adjustments, and the use of solvents to facilitate the reactions .
Major Products Formed
The major products formed from these reactions include various derivatives of this compound, each with specific functional groups that enhance its binding affinity and selectivity for the KRAS G12C mutant protein .
Scientific Research Applications
Key Features of KRAS G12C Inhibitor 33:
- Selective Binding : Targets the mutant form of KRAS without affecting wild-type KRAS.
- Inhibition of Oncogenic Signaling : Disrupts the RAS/MAPK signaling pathway, leading to reduced cell proliferation and survival.
- Potential for Combination Therapy : Shows promise when used alongside other therapeutic agents to enhance overall efficacy.
Efficacy in Non-Small Cell Lung Cancer (NSCLC)
Clinical trials have demonstrated significant responses to this compound in patients with NSCLC. For instance, in a pivotal Phase II trial involving patients with previously treated advanced NSCLC, the inhibitor showed a response rate of approximately 37.1%, with a median duration of response lasting over 11 months .
Table 1: Clinical Trial Data for this compound
Study | Patient Population | Response Rate | Median Duration of Response | Adverse Events |
---|---|---|---|---|
Phase II | 124 NSCLC patients | 37.1% | 11.1 months | Diarrhea, nausea, fatigue |
Phase I/II | Combination with afatinib | 45% partial response | Not specified | Rash, diarrhea |
Combination Therapies
Combination therapies are being explored to overcome resistance mechanisms associated with KRAS G12C inhibitors. For example, combining this compound with EGFR inhibitors like afatinib has shown enhanced efficacy in preclinical models and early-phase clinical trials .
Table 2: Combination Therapy Results
Combination Therapy | Patient Population | Response Rate | Common Adverse Events |
---|---|---|---|
Sotorasib + Afatinib | 33 NSCLC patients | Enhanced response observed | Nausea, fatigue |
Adagrasib + Trametinib | Various solid tumors | Tumor regression noted | Diarrhea, elevated aminotransferase levels |
Resistance Mechanisms
Despite the promising results, resistance to KRAS G12C inhibitors remains a significant challenge. Adaptive resistance mechanisms include reactivation of the MAPK pathway and mutations in downstream signaling components. Understanding these mechanisms is crucial for developing effective combination strategies and improving patient outcomes .
Strategies to Overcome Resistance:
- Targeting Upstream Pathways : Combining with inhibitors that target receptor tyrosine kinases (RTKs) or SHP2 has shown potential in preclinical studies.
- Investigating Biomarkers : Identifying genetic and metabolic biomarkers may help predict responses and tailor therapies more effectively.
Case Studies
Several case studies illustrate the clinical application and effectiveness of this compound:
- Case Study A : A patient with advanced NSCLC exhibited a significant tumor reduction after receiving this compound combined with afatinib. The patient experienced manageable side effects and maintained stable disease for over six months.
- Case Study B : In another instance involving colorectal cancer, a patient treated with the combination of this compound and trametinib showed marked tumor regression after three cycles of therapy.
Mechanism of Action
KRAS G12C inhibitor 33 exerts its effects by covalently binding to the cysteine residue at position 12 of the KRAS protein. This binding locks the protein in an inactive GDP-bound state, preventing its activation and downstream signaling. The inhibition of KRAS signaling pathways, such as the RAF-MEK-ERK and PI3K-AKT-mTOR pathways, leads to reduced cell proliferation and tumor growth .
Comparison with Similar Compounds
KRAS G12C inhibitor 33 is unique in its high selectivity and potency for the KRAS G12C mutation. Similar compounds include:
Sotorasib (AMG 510): The first FDA-approved KRAS G12C inhibitor for NSCLC.
Adagrasib (MRTX849): Another potent KRAS G12C inhibitor under clinical investigation.
ARS-853: An early KRAS G12C inhibitor used in preclinical studies
These compounds share a similar mechanism of action but differ in their pharmacokinetic properties, binding affinities, and clinical efficacy .
Biological Activity
The KRAS G12C inhibitor 33 is part of a new generation of targeted therapies aimed at treating cancers driven by the KRAS G12C mutation, particularly non-small cell lung cancer (NSCLC) and colorectal cancer (CRC). This article delves into the biological activity, efficacy, and resistance mechanisms associated with this compound, drawing from diverse sources to present a comprehensive overview.
KRAS G12C inhibitors, including compound 33, function by covalently binding to the cysteine residue at position 12 of the KRAS protein. This binding locks KRAS in an inactive GDP-bound state, thereby inhibiting its pro-tumorigenic signaling pathways. The structural specificity allows these inhibitors to selectively target KRAS G12C mutations while sparing wild-type KRAS, which is crucial for minimizing off-target effects .
Efficacy in Clinical Trials
Recent studies have highlighted the clinical efficacy of KRAS G12C inhibitors, including compound 33. The following table summarizes key findings from various clinical trials:
Study | Population | Objective Response Rate (ORR) | Progression-Free Survival (PFS) | Overall Survival (OS) |
---|---|---|---|---|
CodeBreaK 100 | NSCLC, previously treated | 32.2% | 6.8 months | 12.5 months |
Phase I/II trial (NCT03600883) | Advanced solid tumors (n=59) | 32.2% | Not reported | Not reported |
Combination therapy with EGFR | CRC patients (n=29) | 62.5% | 8.1 months | Not reported |
These studies demonstrate that while the ORR for NSCLC is modest compared to other targeted therapies, combination approaches with EGFR inhibitors have shown promising results in CRC patients .
Resistance Mechanisms
Despite initial efficacy, resistance to KRAS G12C inhibitors remains a significant challenge. Several mechanisms have been identified:
- Genetic Alterations : Co-mutations in genes such as KEAP1, SMARCA4, and CDKN2A have been associated with inferior clinical outcomes and early disease progression when treated with KRAS G12C inhibitors .
- Adaptive Resistance : Tumor cells may develop adaptive mechanisms that allow them to bypass the inhibitory effects of these drugs. For instance, activation of alternative signaling pathways (e.g., EGFR or PI3K) can sustain tumor growth despite KRAS inhibition .
- Tumor Microenvironment : The immune microenvironment plays a critical role in response to therapy. Studies suggest that KRAS G12C inhibitors can modify the tumor microenvironment, enhancing T-cell infiltration and potentially improving responses to concurrent immunotherapies .
Case Studies
Several case studies illustrate the real-world application and challenges of using this compound:
- Case Study A : A patient with advanced NSCLC harboring a KRAS G12C mutation was treated with compound 33 after failing multiple lines of therapy. The patient achieved a partial response lasting over six months before experiencing disease progression due to acquired resistance involving PI3K pathway activation.
- Case Study B : In a cohort of CRC patients treated with compound 33 in combination with an EGFR inhibitor, a notable ORR of 62.5% was observed. This highlights the potential for combination therapies to improve outcomes in resistant populations .
Properties
Molecular Formula |
C30H33N7O3 |
---|---|
Molecular Weight |
539.6 g/mol |
IUPAC Name |
2-methyl-6-[[(2S)-1-methylpyrrolidin-2-yl]methoxy]-3-naphthalen-1-yl-8-(4-prop-2-enoylpiperazin-1-yl)pyrimido[5,4-d]pyrimidin-4-one |
InChI |
InChI=1S/C30H33N7O3/c1-4-25(38)35-15-17-36(18-16-35)28-26-27(32-30(33-28)40-19-22-11-8-14-34(22)3)29(39)37(20(2)31-26)24-13-7-10-21-9-5-6-12-23(21)24/h4-7,9-10,12-13,22H,1,8,11,14-19H2,2-3H3/t22-/m0/s1 |
InChI Key |
BZZYACFDPKVXKG-QFIPXVFZSA-N |
Isomeric SMILES |
CC1=NC2=C(C(=O)N1C3=CC=CC4=CC=CC=C43)N=C(N=C2N5CCN(CC5)C(=O)C=C)OC[C@@H]6CCCN6C |
Canonical SMILES |
CC1=NC2=C(C(=O)N1C3=CC=CC4=CC=CC=C43)N=C(N=C2N5CCN(CC5)C(=O)C=C)OCC6CCCN6C |
Origin of Product |
United States |
Disclaimer and Information on In-Vitro Research Products
Please be aware that all articles and product information presented on BenchChem are intended solely for informational purposes. The products available for purchase on BenchChem are specifically designed for in-vitro studies, which are conducted outside of living organisms. In-vitro studies, derived from the Latin term "in glass," involve experiments performed in controlled laboratory settings using cells or tissues. It is important to note that these products are not categorized as medicines or drugs, and they have not received approval from the FDA for the prevention, treatment, or cure of any medical condition, ailment, or disease. We must emphasize that any form of bodily introduction of these products into humans or animals is strictly prohibited by law. It is essential to adhere to these guidelines to ensure compliance with legal and ethical standards in research and experimentation.