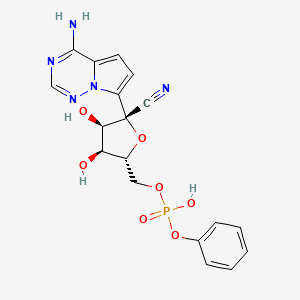
Remdesivir de(ethylbutyl 2-aminopropanoate)
- Click on QUICK INQUIRY to receive a quote from our team of experts.
- With the quality product at a COMPETITIVE price, you can focus more on your research.
Overview
Description
Remdesivir de(ethylbutyl 2-aminopropanoate) is an impurity of Remdesivir, a nucleoside analogue with effective antiviral activity. Remdesivir has been widely studied for its potential in treating various viral infections, including SARS-CoV, MERS-CoV, and SARS-CoV-2 (COVID-19) . The compound Remdesivir de(ethylbutyl 2-aminopropanoate) is of interest due to its structural similarity to Remdesivir and its potential implications in the study of drug metabolism and efficacy.
Preparation Methods
Synthetic Routes and Reaction Conditions
The synthesis of Remdesivir de(ethylbutyl 2-aminopropanoate) involves multiple steps, starting from the basic nucleoside analogue structure. The process typically includes the following steps:
Nucleoside Formation: The initial step involves the formation of the nucleoside analogue through a series of chemical reactions, including glycosylation and phosphorylation.
Esterification: The nucleoside analogue undergoes esterification with ethylbutyl 2-aminopropanoate under controlled conditions to form the desired compound.
Purification: The final product is purified using techniques such as chromatography to ensure the removal of impurities and by-products.
Industrial Production Methods
Industrial production of Remdesivir de(ethylbutyl 2-aminopropanoate) follows similar synthetic routes but on a larger scale. The process involves:
Batch Processing: Large-scale reactors are used to carry out the chemical reactions under controlled conditions.
Quality Control: Rigorous quality control measures are implemented to ensure the consistency and purity of the final product.
Automation: Automated systems are employed to monitor and control the reaction parameters, ensuring efficiency and safety.
Chemical Reactions Analysis
Types of Reactions
Remdesivir de(ethylbutyl 2-aminopropanoate) undergoes various chemical reactions, including:
Oxidation: The compound can undergo oxidation reactions, leading to the formation of oxidized derivatives.
Reduction: Reduction reactions can convert the compound into reduced forms with different chemical properties.
Substitution: Substitution reactions can occur, where functional groups in the compound are replaced with other groups.
Common Reagents and Conditions
Oxidation: Common oxidizing agents include hydrogen peroxide and potassium permanganate.
Reduction: Reducing agents such as sodium borohydride and lithium aluminum hydride are used.
Substitution: Various reagents, including halogens and nucleophiles, are employed under specific conditions to achieve substitution reactions.
Major Products Formed
Scientific Research Applications
Remdesivir de(ethylbutyl 2-aminopropanoate) has several scientific research applications:
Chemistry: The compound is used in the study of nucleoside analogues and their chemical properties.
Biology: It is employed in research on viral replication and the development of antiviral drugs.
Medicine: The compound is studied for its potential therapeutic effects and its role in drug metabolism.
Industry: It is used in the development and testing of pharmaceutical formulations and drug delivery systems.
Mechanism of Action
The mechanism of action of Remdesivir de(ethylbutyl 2-aminopropanoate) involves its interaction with viral RNA polymerase. The compound acts as a chain terminator, inhibiting the replication of viral RNA. This mechanism is similar to that of Remdesivir, which targets the RNA-dependent RNA polymerase of RNA viruses . The molecular targets and pathways involved include the inhibition of viral RNA synthesis and the disruption of viral replication.
Comparison with Similar Compounds
Similar Compounds
Remdesivir: The parent compound with broad-spectrum antiviral activity.
GS-441524: A metabolite of Remdesivir with similar antiviral properties.
Favipiravir: Another nucleoside analogue with antiviral activity against RNA viruses.
Uniqueness
Remdesivir de(ethylbutyl 2-aminopropanoate) is unique due to its specific structural modifications, which may influence its pharmacokinetic and pharmacodynamic properties. Its study provides insights into the metabolism and efficacy of nucleoside analogues, contributing to the development of more effective antiviral therapies.
Properties
Molecular Formula |
C18H18N5O7P |
---|---|
Molecular Weight |
447.3 g/mol |
IUPAC Name |
[(2R,3S,4R,5R)-5-(4-aminopyrrolo[2,1-f][1,2,4]triazin-7-yl)-5-cyano-3,4-dihydroxyoxolan-2-yl]methyl phenyl hydrogen phosphate |
InChI |
InChI=1S/C18H18N5O7P/c19-9-18(14-7-6-12-17(20)21-10-22-23(12)14)16(25)15(24)13(29-18)8-28-31(26,27)30-11-4-2-1-3-5-11/h1-7,10,13,15-16,24-25H,8H2,(H,26,27)(H2,20,21,22)/t13-,15-,16-,18+/m1/s1 |
InChI Key |
MRXDDYZPVOXRJV-IIVZCXTMSA-N |
Isomeric SMILES |
C1=CC=C(C=C1)OP(=O)(O)OC[C@@H]2[C@H]([C@H]([C@](O2)(C#N)C3=CC=C4N3N=CN=C4N)O)O |
Canonical SMILES |
C1=CC=C(C=C1)OP(=O)(O)OCC2C(C(C(O2)(C#N)C3=CC=C4N3N=CN=C4N)O)O |
Origin of Product |
United States |
Disclaimer and Information on In-Vitro Research Products
Please be aware that all articles and product information presented on BenchChem are intended solely for informational purposes. The products available for purchase on BenchChem are specifically designed for in-vitro studies, which are conducted outside of living organisms. In-vitro studies, derived from the Latin term "in glass," involve experiments performed in controlled laboratory settings using cells or tissues. It is important to note that these products are not categorized as medicines or drugs, and they have not received approval from the FDA for the prevention, treatment, or cure of any medical condition, ailment, or disease. We must emphasize that any form of bodily introduction of these products into humans or animals is strictly prohibited by law. It is essential to adhere to these guidelines to ensure compliance with legal and ethical standards in research and experimentation.