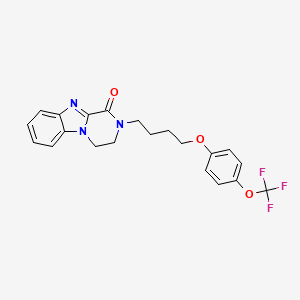
mGluR2 modulator 1
- Click on QUICK INQUIRY to receive a quote from our team of experts.
- With the quality product at a COMPETITIVE price, you can focus more on your research.
Overview
Description
mGluR2 modulator 1 is a potent and selective positive allosteric modulator of the metabotropic glutamate receptor subtype 2 (mGluR2). This compound has shown promise in various scientific research applications, particularly in the fields of neuroscience and pharmacology. mGluR2 is a G-protein-coupled receptor that plays a crucial role in modulating synaptic transmission and neuronal excitability in the central nervous system.
Preparation Methods
The synthesis of mGluR2 modulator 1 involves several steps, including the preparation of key intermediates and the final coupling reactions. The synthetic route typically starts with commercially available starting materials, which undergo a series of chemical transformations such as cyclization, Suzuki coupling, and reduction reactions. The reaction conditions often involve the use of palladium catalysts, organic solvents, and controlled temperatures to achieve high yields and purity of the final product .
Chemical Reactions Analysis
mGluR2 modulator 1 undergoes various chemical reactions, including:
Oxidation: This reaction involves the addition of oxygen or the removal of hydrogen atoms, often using oxidizing agents such as potassium permanganate or hydrogen peroxide.
Reduction: This reaction involves the addition of hydrogen atoms or the removal of oxygen atoms, typically using reducing agents like sodium borohydride or lithium aluminum hydride.
Substitution: This reaction involves the replacement of one functional group with another, often using reagents such as halogens or nucleophiles. The major products formed from these reactions depend on the specific reagents and conditions used, but they generally include various derivatives of the original compound
Scientific Research Applications
mGluR2 modulator 1 has a wide range of scientific research applications, including:
Neuroscience: It is used to study the role of mGluR2 in modulating synaptic transmission and neuronal excitability.
Pharmacology: It is used to develop new therapeutic agents targeting mGluR2 for the treatment of various neurological and psychiatric conditions.
Mechanism of Action
mGluR2 modulator 1 exerts its effects by binding to the allosteric site of the mGluR2 receptor, which enhances the receptor’s response to its natural ligand, glutamate. This positive allosteric modulation leads to increased activation of the receptor, resulting in the inhibition of neurotransmitter release and modulation of synaptic transmission. The molecular targets and pathways involved include the inhibition of adenylyl cyclase activity, reduction of cyclic AMP levels, and modulation of downstream signaling pathways .
Comparison with Similar Compounds
mGluR2 modulator 1 can be compared with other similar compounds, such as:
Biphenyl-indanone A (BINA): Another positive allosteric modulator of mGluR2, which has shown antipsychotic and anxiolytic effects in preclinical studies.
LY-487,379: A structurally similar compound that also acts as a positive allosteric modulator of mGluR2.
CBiPES: Another mGluR2 positive allosteric modulator with a similar chemical scaffold. The uniqueness of this compound lies in its high potency, selectivity, and ability to penetrate the blood-brain barrier, making it a valuable tool for scientific research and potential therapeutic applications.
Properties
Molecular Formula |
C21H20F3N3O3 |
---|---|
Molecular Weight |
419.4 g/mol |
IUPAC Name |
2-[4-[4-(trifluoromethoxy)phenoxy]butyl]-3,4-dihydropyrazino[1,2-a]benzimidazol-1-one |
InChI |
InChI=1S/C21H20F3N3O3/c22-21(23,24)30-16-9-7-15(8-10-16)29-14-4-3-11-26-12-13-27-18-6-2-1-5-17(18)25-19(27)20(26)28/h1-2,5-10H,3-4,11-14H2 |
InChI Key |
QRCUMXUPIXTGPF-UHFFFAOYSA-N |
Canonical SMILES |
C1CN2C3=CC=CC=C3N=C2C(=O)N1CCCCOC4=CC=C(C=C4)OC(F)(F)F |
Origin of Product |
United States |
Disclaimer and Information on In-Vitro Research Products
Please be aware that all articles and product information presented on BenchChem are intended solely for informational purposes. The products available for purchase on BenchChem are specifically designed for in-vitro studies, which are conducted outside of living organisms. In-vitro studies, derived from the Latin term "in glass," involve experiments performed in controlled laboratory settings using cells or tissues. It is important to note that these products are not categorized as medicines or drugs, and they have not received approval from the FDA for the prevention, treatment, or cure of any medical condition, ailment, or disease. We must emphasize that any form of bodily introduction of these products into humans or animals is strictly prohibited by law. It is essential to adhere to these guidelines to ensure compliance with legal and ethical standards in research and experimentation.