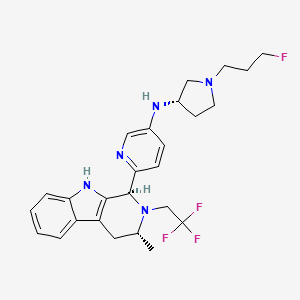
Estrogen receptor antagonist 2
- Click on QUICK INQUIRY to receive a quote from our team of experts.
- With the quality product at a COMPETITIVE price, you can focus more on your research.
Overview
Description
Estrogen receptor antagonist 2 is a compound that inhibits the activity of estrogen receptors, which are proteins that mediate the effects of estrogen hormones in the body. Estrogen receptors are involved in various physiological processes, including cell growth, differentiation, and reproduction. This compound is particularly significant in the treatment of estrogen receptor-positive breast cancer, where it blocks the proliferative effects of estrogen on cancer cells .
Preparation Methods
Synthetic Routes and Reaction Conditions: The synthesis of estrogen receptor antagonist 2 involves multiple steps, including the formation of key intermediates and their subsequent functionalizationCommon reaction conditions include the use of organic solvents, catalysts, and controlled temperatures to achieve high yields and purity .
Industrial Production Methods: Industrial production of this compound involves scaling up the laboratory synthesis to a larger scale. This requires optimization of reaction conditions, purification processes, and quality control measures to ensure consistency and safety. Techniques such as high-performance liquid chromatography and mass spectrometry are used to monitor the production process and verify the final product’s quality .
Chemical Reactions Analysis
Types of Reactions: Estrogen receptor antagonist 2 undergoes various chemical reactions, including:
Oxidation: This reaction involves the addition of oxygen or the removal of hydrogen, often using oxidizing agents such as hydrogen peroxide or potassium permanganate.
Reduction: This reaction involves the addition of hydrogen or the removal of oxygen, typically using reducing agents like sodium borohydride or lithium aluminum hydride.
Common Reagents and Conditions:
Oxidation: Hydrogen peroxide, potassium permanganate, and other oxidizing agents.
Reduction: Sodium borohydride, lithium aluminum hydride, and other reducing agents.
Substitution: Nucleophiles such as halides, amines, and electrophiles like alkyl halides.
Major Products: The major products formed from these reactions depend on the specific functional groups involved and the reaction conditions. For example, oxidation may yield hydroxylated derivatives, while reduction may produce deoxygenated compounds .
Scientific Research Applications
Estrogen receptor antagonist 2 has a wide range of scientific research applications, including:
Chemistry: Used as a tool to study the structure and function of estrogen receptors and their interactions with other molecules.
Biology: Helps in understanding the role of estrogen receptors in cellular processes and their regulation.
Medicine: Plays a crucial role in the treatment of estrogen receptor-positive breast cancer by inhibiting the growth of cancer cells.
Mechanism of Action
Estrogen receptor antagonist 2 exerts its effects by binding to estrogen receptors and preventing the activation of downstream signaling pathways. It competes with endogenous estrogens for binding to the receptor’s ligand-binding domain, thereby blocking the receptor’s ability to regulate gene expression. This inhibition leads to a decrease in cell proliferation and survival, particularly in estrogen receptor-positive breast cancer cells .
Comparison with Similar Compounds
Fulvestrant: A selective estrogen receptor degrader that binds to estrogen receptors and promotes their degradation.
Tamoxifen: A selective estrogen receptor modulator that acts as an antagonist in breast tissue but can have agonist effects in other tissues.
Elacestrant: Another estrogen receptor antagonist that is orally bioavailable and used in the treatment of advanced breast cancer.
Uniqueness: Estrogen receptor antagonist 2 is unique in its ability to completely block estrogen-induced transcriptional activity without any agonist effects. It also demonstrates superior pharmacokinetic properties, including oral bioavailability and brain penetrance, making it a promising candidate for the treatment of estrogen receptor-positive breast cancer .
Properties
Molecular Formula |
C26H31F4N5 |
---|---|
Molecular Weight |
489.6 g/mol |
IUPAC Name |
N-[(3S)-1-(3-fluoropropyl)pyrrolidin-3-yl]-6-[(1S,3R)-3-methyl-2-(2,2,2-trifluoroethyl)-1,3,4,9-tetrahydropyrido[3,4-b]indol-1-yl]pyridin-3-amine |
InChI |
InChI=1S/C26H31F4N5/c1-17-13-21-20-5-2-3-6-22(20)33-24(21)25(35(17)16-26(28,29)30)23-8-7-18(14-31-23)32-19-9-12-34(15-19)11-4-10-27/h2-3,5-8,14,17,19,25,32-33H,4,9-13,15-16H2,1H3/t17-,19+,25-/m1/s1 |
InChI Key |
GRCKMGXTYAMEJC-VDABOFBKSA-N |
Isomeric SMILES |
C[C@@H]1CC2=C([C@H](N1CC(F)(F)F)C3=NC=C(C=C3)N[C@H]4CCN(C4)CCCF)NC5=CC=CC=C25 |
Canonical SMILES |
CC1CC2=C(C(N1CC(F)(F)F)C3=NC=C(C=C3)NC4CCN(C4)CCCF)NC5=CC=CC=C25 |
Origin of Product |
United States |
Disclaimer and Information on In-Vitro Research Products
Please be aware that all articles and product information presented on BenchChem are intended solely for informational purposes. The products available for purchase on BenchChem are specifically designed for in-vitro studies, which are conducted outside of living organisms. In-vitro studies, derived from the Latin term "in glass," involve experiments performed in controlled laboratory settings using cells or tissues. It is important to note that these products are not categorized as medicines or drugs, and they have not received approval from the FDA for the prevention, treatment, or cure of any medical condition, ailment, or disease. We must emphasize that any form of bodily introduction of these products into humans or animals is strictly prohibited by law. It is essential to adhere to these guidelines to ensure compliance with legal and ethical standards in research and experimentation.