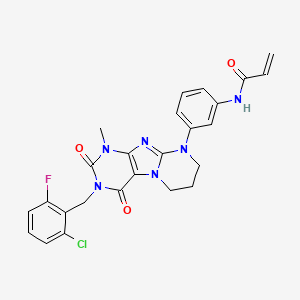
KRAS G12C inhibitor 30
- Click on QUICK INQUIRY to receive a quote from our team of experts.
- With the quality product at a COMPETITIVE price, you can focus more on your research.
Overview
Description
KRAS G12C inhibitor 30 is a compound designed to target the KRAS G12C mutation, a common mutation in the KRAS gene found in various cancers, including non-small cell lung cancer (NSCLC), colorectal cancer, and pancreatic cancer . This mutation results in the substitution of glycine with cysteine at position 12 of the KRAS protein, leading to uncontrolled cell growth and cancer progression . KRAS G12C inhibitors, such as this compound, specifically bind to the mutant cysteine residue, thereby inhibiting the oncogenic activity of KRAS .
Preparation Methods
Synthetic Routes and Reaction Conditions: The synthesis of KRAS G12C inhibitor 30 involves multiple steps, including the formation of key intermediates and the final coupling reactions . The synthetic route typically starts with the preparation of a core scaffold, followed by functionalization to introduce the desired substituents. Common reaction conditions include the use of organic solvents, catalysts, and controlled temperatures to achieve high yields and purity .
Industrial Production Methods: Industrial production of this compound involves scaling up the laboratory synthesis to a larger scale while maintaining the quality and consistency of the product . This process includes optimizing reaction conditions, purification methods, and quality control measures to ensure the final product meets regulatory standards .
Chemical Reactions Analysis
Types of Reactions: KRAS G12C inhibitor 30 undergoes various chemical reactions, including covalent binding to the thiol group of the cysteine residue in the KRAS G12C mutant . This covalent modification traps KRAS in an inactive GDP-bound state, preventing its oncogenic activity .
Common Reagents and Conditions: Common reagents used in the synthesis and reactions of this compound include organic solvents, catalysts, and protective groups to ensure selective reactions . Reaction conditions often involve controlled temperatures, inert atmospheres, and specific pH levels to achieve optimal results .
Major Products Formed: The major product formed from the reaction of this compound with the KRAS G12C mutant is the covalently modified KRAS protein, which is rendered inactive and unable to promote cancer cell growth .
Scientific Research Applications
KRAS G12C inhibitor 30 has a wide range of scientific research applications, particularly in the fields of chemistry, biology, medicine, and industry . In chemistry, it serves as a model compound for studying covalent inhibitors and their interactions with target proteins . In biology, it is used to investigate the role of KRAS mutations in cancer progression and to develop new therapeutic strategies . In medicine, this compound is being evaluated in clinical trials for its efficacy in treating cancers harboring the KRAS G12C mutation .
Mechanism of Action
KRAS G12C inhibitor 30 exerts its effects by covalently binding to the cysteine residue at position 12 of the KRAS G12C mutant . This binding traps KRAS in an inactive GDP-bound state, preventing its activation and subsequent signaling through downstream pathways such as the RAF-MEK-MAPK and PI3K-AKT-mTOR pathways . By inhibiting these pathways, this compound effectively blocks the oncogenic activity of KRAS and reduces cancer cell proliferation .
Comparison with Similar Compounds
Compared to these inhibitors, KRAS G12C inhibitor 30 may have unique structural features or binding properties that enhance its efficacy or selectivity . For example, it may exhibit improved binding affinity to the KRAS G12C mutant or better pharmacokinetic properties . Similar compounds in this class include divarasib and other investigational KRAS G12C inhibitors currently in clinical trials .
Biological Activity
The KRAS G12C mutation is a significant oncogenic driver found predominantly in non-small cell lung cancer (NSCLC) and other malignancies. The development of KRAS G12C inhibitors has revolutionized the treatment landscape for patients with this mutation. Among these inhibitors, KRAS G12C inhibitor 30 has emerged as a promising candidate, demonstrating unique biological activity that warrants detailed exploration.
KRAS proteins function as molecular switches that cycle between an active GTP-bound state and an inactive GDP-bound state. The G12C mutation alters this cycling, leading to persistent activation and oncogenic signaling. KRAS G12C inhibitors, including inhibitor 30, selectively bind to the GDP-bound form of KRAS, thereby inhibiting its activity. This selective binding is crucial as it allows for targeted disruption of KRAS signaling pathways while minimizing effects on wild-type RAS proteins.
Comparative Potency and Efficacy
In preclinical studies, this compound has shown substantial potency compared to existing inhibitors. The following table summarizes the IC50 values for various KRAS G12C inhibitors:
Inhibitor | IC50 (nM) |
---|---|
ARS-853 | 5899 |
ARS-1620 | 692 |
Sotorasib | 35 |
Adagrasib | 78 |
This compound | 0.6 |
These results indicate that this compound exhibits a significantly lower IC50 value, suggesting higher potency in inhibiting active KRAS compared to its predecessors .
Preclinical Studies
In vitro studies using NSCLC cell lines harboring the KRAS G12C mutation have demonstrated that inhibitor 30 effectively reduces cellular levels of active KRAS in a dose-dependent manner. For instance, treatment with inhibitor 30 resulted in a rapid depletion of active KRAS within hours, even in the presence of growth factors, which is a notable advancement over earlier generation inhibitors .
Clinical Insights
Early-phase clinical trials have begun to evaluate the safety and pharmacokinetics of this compound in patients with advanced solid tumors. Initial results indicate promising systemic activity across various dose cohorts, with objective response rates comparable to or exceeding those observed with sotorasib and adagrasib .
Case Study: Patient Response
A case study involving a patient with heavily pre-treated NSCLC showed a significant reduction in tumor size following treatment with this compound. The patient had previously failed multiple lines of therapy but exhibited a partial response after two cycles of treatment, highlighting the potential for this compound in refractory cases .
Resistance Mechanisms
Despite the efficacy of KRAS G12C inhibitors, resistance mechanisms remain a challenge. Studies have shown that some tumors develop secondary mutations or activate alternative signaling pathways that bypass KRAS dependency. Combination therapies targeting these pathways are currently under investigation to enhance the overall therapeutic efficacy of KRAS G12C inhibitors .
Properties
Molecular Formula |
C25H22ClFN6O3 |
---|---|
Molecular Weight |
508.9 g/mol |
IUPAC Name |
N-[3-[3-[(2-chloro-6-fluorophenyl)methyl]-1-methyl-2,4-dioxo-7,8-dihydro-6H-purino[7,8-a]pyrimidin-9-yl]phenyl]prop-2-enamide |
InChI |
InChI=1S/C25H22ClFN6O3/c1-3-20(34)28-15-7-4-8-16(13-15)31-11-6-12-32-21-22(29-24(31)32)30(2)25(36)33(23(21)35)14-17-18(26)9-5-10-19(17)27/h3-5,7-10,13H,1,6,11-12,14H2,2H3,(H,28,34) |
InChI Key |
FYTIAUJXQNHTOR-UHFFFAOYSA-N |
Canonical SMILES |
CN1C2=C(C(=O)N(C1=O)CC3=C(C=CC=C3Cl)F)N4CCCN(C4=N2)C5=CC=CC(=C5)NC(=O)C=C |
Origin of Product |
United States |
Disclaimer and Information on In-Vitro Research Products
Please be aware that all articles and product information presented on BenchChem are intended solely for informational purposes. The products available for purchase on BenchChem are specifically designed for in-vitro studies, which are conducted outside of living organisms. In-vitro studies, derived from the Latin term "in glass," involve experiments performed in controlled laboratory settings using cells or tissues. It is important to note that these products are not categorized as medicines or drugs, and they have not received approval from the FDA for the prevention, treatment, or cure of any medical condition, ailment, or disease. We must emphasize that any form of bodily introduction of these products into humans or animals is strictly prohibited by law. It is essential to adhere to these guidelines to ensure compliance with legal and ethical standards in research and experimentation.