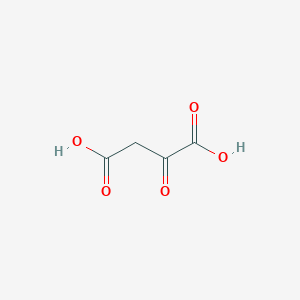
Oxalacetic acid
Overview
Description
Mechanism of Action
- Role : It serves as an intermediate in several biochemical reactions, influencing energy production, biosynthesis, and cellular homeostasis .
- Inhibition of Complex II : Oxaloacetic acid acts as a potent inhibitor of complex II (succinate dehydrogenase) in the electron transport chain .
Target of Action
Mode of Action
Biochemical Pathways
Pharmacokinetics
Biochemical Analysis
Biochemical Properties
Oxalacetic acid is an intermediate of the citric acid cycle, where it reacts with acetyl-CoA to form citrate, catalyzed by citrate synthase . It is also involved in gluconeogenesis, the urea cycle, the glyoxylate cycle, amino acid synthesis, and fatty acid synthesis . This compound undergoes successive deprotonations to give the dianion, and at high pH, the enolizable proton is ionized .
Cellular Effects
This compound plays a crucial role in regulating glucose metabolism and promoting insulin sensitivity, which may help regulate blood sugar levels and support weight management . Furthermore, research suggests that this compound may have antioxidant and neuroprotective properties, making it a topic of interest in the field of neurodegenerative diseases .
Molecular Mechanism
This compound exerts its effects at the molecular level through various mechanisms. It is a key intermediate in the citric acid cycle, where it reacts with acetyl-CoA to form citrate, catalyzed by citrate synthase . It also arises from the condensation of pyruvate with carbonic acid, driven by the hydrolysis of ATP . This compound can also arise from trans- or de-amination of aspartic acid .
Metabolic Pathways
This compound is involved in several metabolic pathways. It is an intermediate in the citric acid cycle, where it reacts with acetyl-CoA to form citrate . It is also involved in gluconeogenesis, the urea cycle, the glyoxylate cycle, amino acid synthesis, and fatty acid synthesis .
Preparation Methods
Synthetic Routes and Reaction Conditions: Oxaloacetic acid can be synthesized through the oxidation of L-malate, catalyzed by malate dehydrogenase in the citric acid cycle . Another method involves the condensation of pyruvate with carbonic acid, driven by the hydrolysis of adenosine triphosphate (ATP) . This process occurs in the mesophyll of plants and is catalyzed by phosphoenolpyruvate carboxylase .
Industrial Production Methods: Industrial production of oxaloacetic acid typically involves the microbial fermentation of carbohydrates. Specific strains of bacteria or yeast are used to convert glucose or other sugars into oxaloacetic acid through a series of enzymatic reactions. The product is then purified using techniques such as crystallization or chromatography .
Chemical Reactions Analysis
Types of Reactions: Oxaloacetic acid undergoes various chemical reactions, including:
Oxidation: Oxaloacetic acid can be oxidized to form carbon dioxide and water.
Reduction: It can be reduced to malate by malate dehydrogenase.
Decarboxylation: Oxaloacetic acid can undergo decarboxylation to form pyruvate.
Common Reagents and Conditions:
Oxidation: Catalyzed by enzymes such as malate dehydrogenase.
Reduction: Catalyzed by enzymes such as malate dehydrogenase.
Decarboxylation: Catalyzed by enzymes such as oxaloacetate decarboxylase.
Major Products:
Oxidation: Carbon dioxide and water.
Reduction: Malate.
Decarboxylation: Pyruvate.
Scientific Research Applications
Oxaloacetic acid has several important applications in scientific research:
Chemistry: Used as a reagent in various chemical reactions and as a standard in analytical chemistry.
Comparison with Similar Compounds
Pyruvate: Another key intermediate in metabolic pathways, involved in glycolysis and the citric acid cycle.
Malate: An intermediate in the citric acid cycle, formed by the reduction of oxaloacetic acid.
Fumarate: Another intermediate in the citric acid cycle, formed by the hydration of fumaric acid.
Uniqueness: Oxaloacetic acid is unique due to its role in multiple metabolic pathways, including the citric acid cycle, gluconeogenesis, and fatty acid biosynthesis. Its ability to act as a substrate for various enzymes and its involvement in energy metabolism make it a critical compound in both biological and industrial contexts .
Properties
IUPAC Name |
2-oxobutanedioic acid | |
---|---|---|
Source | PubChem | |
URL | https://pubchem.ncbi.nlm.nih.gov | |
Description | Data deposited in or computed by PubChem | |
InChI |
InChI=1S/C4H4O5/c5-2(4(8)9)1-3(6)7/h1H2,(H,6,7)(H,8,9) | |
Source | PubChem | |
URL | https://pubchem.ncbi.nlm.nih.gov | |
Description | Data deposited in or computed by PubChem | |
InChI Key |
KHPXUQMNIQBQEV-UHFFFAOYSA-N | |
Source | PubChem | |
URL | https://pubchem.ncbi.nlm.nih.gov | |
Description | Data deposited in or computed by PubChem | |
Canonical SMILES |
C(C(=O)C(=O)O)C(=O)O | |
Source | PubChem | |
URL | https://pubchem.ncbi.nlm.nih.gov | |
Description | Data deposited in or computed by PubChem | |
Molecular Formula |
C4H4O5 | |
Source | PubChem | |
URL | https://pubchem.ncbi.nlm.nih.gov | |
Description | Data deposited in or computed by PubChem | |
DSSTOX Substance ID |
DTXSID8021646 | |
Record name | 2-Oxobutanedioic acid | |
Source | EPA DSSTox | |
URL | https://comptox.epa.gov/dashboard/DTXSID8021646 | |
Description | DSSTox provides a high quality public chemistry resource for supporting improved predictive toxicology. | |
Molecular Weight |
132.07 g/mol | |
Source | PubChem | |
URL | https://pubchem.ncbi.nlm.nih.gov | |
Description | Data deposited in or computed by PubChem | |
Physical Description |
Off-white powder; [Alfa Aesar MSDS], Solid | |
Record name | Oxalacetic acid | |
Source | Haz-Map, Information on Hazardous Chemicals and Occupational Diseases | |
URL | https://haz-map.com/Agents/16302 | |
Description | Haz-Map® is an occupational health database designed for health and safety professionals and for consumers seeking information about the adverse effects of workplace exposures to chemical and biological agents. | |
Explanation | Copyright (c) 2022 Haz-Map(R). All rights reserved. Unless otherwise indicated, all materials from Haz-Map are copyrighted by Haz-Map(R). No part of these materials, either text or image may be used for any purpose other than for personal use. Therefore, reproduction, modification, storage in a retrieval system or retransmission, in any form or by any means, electronic, mechanical or otherwise, for reasons other than personal use, is strictly prohibited without prior written permission. | |
Record name | Oxalacetic acid | |
Source | Human Metabolome Database (HMDB) | |
URL | http://www.hmdb.ca/metabolites/HMDB0000223 | |
Description | The Human Metabolome Database (HMDB) is a freely available electronic database containing detailed information about small molecule metabolites found in the human body. | |
Explanation | HMDB is offered to the public as a freely available resource. Use and re-distribution of the data, in whole or in part, for commercial purposes requires explicit permission of the authors and explicit acknowledgment of the source material (HMDB) and the original publication (see the HMDB citing page). We ask that users who download significant portions of the database cite the HMDB paper in any resulting publications. | |
Solubility |
134 mg/mL | |
Record name | Oxalacetic acid | |
Source | Human Metabolome Database (HMDB) | |
URL | http://www.hmdb.ca/metabolites/HMDB0000223 | |
Description | The Human Metabolome Database (HMDB) is a freely available electronic database containing detailed information about small molecule metabolites found in the human body. | |
Explanation | HMDB is offered to the public as a freely available resource. Use and re-distribution of the data, in whole or in part, for commercial purposes requires explicit permission of the authors and explicit acknowledgment of the source material (HMDB) and the original publication (see the HMDB citing page). We ask that users who download significant portions of the database cite the HMDB paper in any resulting publications. | |
CAS No. |
328-42-7 | |
Record name | Oxaloacetic acid | |
Source | CAS Common Chemistry | |
URL | https://commonchemistry.cas.org/detail?cas_rn=328-42-7 | |
Description | CAS Common Chemistry is an open community resource for accessing chemical information. Nearly 500,000 chemical substances from CAS REGISTRY cover areas of community interest, including common and frequently regulated chemicals, and those relevant to high school and undergraduate chemistry classes. This chemical information, curated by our expert scientists, is provided in alignment with our mission as a division of the American Chemical Society. | |
Explanation | The data from CAS Common Chemistry is provided under a CC-BY-NC 4.0 license, unless otherwise stated. | |
Record name | Oxalacetic acid | |
Source | ChemIDplus | |
URL | https://pubchem.ncbi.nlm.nih.gov/substance/?source=chemidplus&sourceid=0000328427 | |
Description | ChemIDplus is a free, web search system that provides access to the structure and nomenclature authority files used for the identification of chemical substances cited in National Library of Medicine (NLM) databases, including the TOXNET system. | |
Record name | Oxaloacetic acid | |
Source | DrugBank | |
URL | https://www.drugbank.ca/drugs/DB16921 | |
Description | The DrugBank database is a unique bioinformatics and cheminformatics resource that combines detailed drug (i.e. chemical, pharmacological and pharmaceutical) data with comprehensive drug target (i.e. sequence, structure, and pathway) information. | |
Explanation | Creative Common's Attribution-NonCommercial 4.0 International License (http://creativecommons.org/licenses/by-nc/4.0/legalcode) | |
Record name | oxalacetic acid | |
Source | DTP/NCI | |
URL | https://dtp.cancer.gov/dtpstandard/servlet/dwindex?searchtype=NSC&outputformat=html&searchlist=77688 | |
Description | The NCI Development Therapeutics Program (DTP) provides services and resources to the academic and private-sector research communities worldwide to facilitate the discovery and development of new cancer therapeutic agents. | |
Explanation | Unless otherwise indicated, all text within NCI products is free of copyright and may be reused without our permission. Credit the National Cancer Institute as the source. | |
Record name | Butanedioic acid, 2-oxo- | |
Source | EPA Chemicals under the TSCA | |
URL | https://www.epa.gov/chemicals-under-tsca | |
Description | EPA Chemicals under the Toxic Substances Control Act (TSCA) collection contains information on chemicals and their regulations under TSCA, including non-confidential content from the TSCA Chemical Substance Inventory and Chemical Data Reporting. | |
Record name | 2-Oxobutanedioic acid | |
Source | EPA DSSTox | |
URL | https://comptox.epa.gov/dashboard/DTXSID8021646 | |
Description | DSSTox provides a high quality public chemistry resource for supporting improved predictive toxicology. | |
Record name | Oxalacetic acid | |
Source | European Chemicals Agency (ECHA) | |
URL | https://echa.europa.eu/substance-information/-/substanceinfo/100.005.755 | |
Description | The European Chemicals Agency (ECHA) is an agency of the European Union which is the driving force among regulatory authorities in implementing the EU's groundbreaking chemicals legislation for the benefit of human health and the environment as well as for innovation and competitiveness. | |
Explanation | Use of the information, documents and data from the ECHA website is subject to the terms and conditions of this Legal Notice, and subject to other binding limitations provided for under applicable law, the information, documents and data made available on the ECHA website may be reproduced, distributed and/or used, totally or in part, for non-commercial purposes provided that ECHA is acknowledged as the source: "Source: European Chemicals Agency, http://echa.europa.eu/". Such acknowledgement must be included in each copy of the material. ECHA permits and encourages organisations and individuals to create links to the ECHA website under the following cumulative conditions: Links can only be made to webpages that provide a link to the Legal Notice page. | |
Record name | OXALOACETIC ACID | |
Source | FDA Global Substance Registration System (GSRS) | |
URL | https://gsrs.ncats.nih.gov/ginas/app/beta/substances/2F399MM81J | |
Description | The FDA Global Substance Registration System (GSRS) enables the efficient and accurate exchange of information on what substances are in regulated products. Instead of relying on names, which vary across regulatory domains, countries, and regions, the GSRS knowledge base makes it possible for substances to be defined by standardized, scientific descriptions. | |
Explanation | Unless otherwise noted, the contents of the FDA website (www.fda.gov), both text and graphics, are not copyrighted. They are in the public domain and may be republished, reprinted and otherwise used freely by anyone without the need to obtain permission from FDA. Credit to the U.S. Food and Drug Administration as the source is appreciated but not required. | |
Record name | Oxalacetic acid | |
Source | Human Metabolome Database (HMDB) | |
URL | http://www.hmdb.ca/metabolites/HMDB0000223 | |
Description | The Human Metabolome Database (HMDB) is a freely available electronic database containing detailed information about small molecule metabolites found in the human body. | |
Explanation | HMDB is offered to the public as a freely available resource. Use and re-distribution of the data, in whole or in part, for commercial purposes requires explicit permission of the authors and explicit acknowledgment of the source material (HMDB) and the original publication (see the HMDB citing page). We ask that users who download significant portions of the database cite the HMDB paper in any resulting publications. | |
Melting Point |
161 °C | |
Record name | Oxalacetic acid | |
Source | Human Metabolome Database (HMDB) | |
URL | http://www.hmdb.ca/metabolites/HMDB0000223 | |
Description | The Human Metabolome Database (HMDB) is a freely available electronic database containing detailed information about small molecule metabolites found in the human body. | |
Explanation | HMDB is offered to the public as a freely available resource. Use and re-distribution of the data, in whole or in part, for commercial purposes requires explicit permission of the authors and explicit acknowledgment of the source material (HMDB) and the original publication (see the HMDB citing page). We ask that users who download significant portions of the database cite the HMDB paper in any resulting publications. | |
Retrosynthesis Analysis
AI-Powered Synthesis Planning: Our tool employs the Template_relevance Pistachio, Template_relevance Bkms_metabolic, Template_relevance Pistachio_ringbreaker, Template_relevance Reaxys, Template_relevance Reaxys_biocatalysis model, leveraging a vast database of chemical reactions to predict feasible synthetic routes.
One-Step Synthesis Focus: Specifically designed for one-step synthesis, it provides concise and direct routes for your target compounds, streamlining the synthesis process.
Accurate Predictions: Utilizing the extensive PISTACHIO, BKMS_METABOLIC, PISTACHIO_RINGBREAKER, REAXYS, REAXYS_BIOCATALYSIS database, our tool offers high-accuracy predictions, reflecting the latest in chemical research and data.
Strategy Settings
Precursor scoring | Relevance Heuristic |
---|---|
Min. plausibility | 0.01 |
Model | Template_relevance |
Template Set | Pistachio/Bkms_metabolic/Pistachio_ringbreaker/Reaxys/Reaxys_biocatalysis |
Top-N result to add to graph | 6 |
Feasible Synthetic Routes
Disclaimer and Information on In-Vitro Research Products
Please be aware that all articles and product information presented on BenchChem are intended solely for informational purposes. The products available for purchase on BenchChem are specifically designed for in-vitro studies, which are conducted outside of living organisms. In-vitro studies, derived from the Latin term "in glass," involve experiments performed in controlled laboratory settings using cells or tissues. It is important to note that these products are not categorized as medicines or drugs, and they have not received approval from the FDA for the prevention, treatment, or cure of any medical condition, ailment, or disease. We must emphasize that any form of bodily introduction of these products into humans or animals is strictly prohibited by law. It is essential to adhere to these guidelines to ensure compliance with legal and ethical standards in research and experimentation.