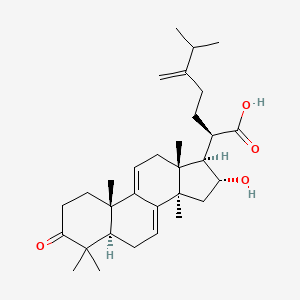
Polyporenic acid C
Overview
Description
Synthesis Analysis
The synthesis of polyporenic acid C, like other triterpenic acids, involves complex biochemical pathways within fungi, particularly in wood-rotting species such as Polyporus officinalis. These compounds are typically derived from squalene, a precursor in the sterol biosynthesis pathway, through a series of enzymatic transformations leading to the characteristic triterpenic structure.
Molecular Structure Analysis
This compound is characterized by a triterpenoid skeleton, which is a common feature among fungal metabolites. This structure includes multiple ring systems, often with a pentacyclic framework, and functional groups such as hydroxyls, which are crucial for its bioactivity. The exact structure can vary slightly depending on the species from which it is isolated and the specific biosynthetic route.
Chemical Reactions and Properties
Triterpenic acids, including this compound, can undergo various chemical reactions due to their functional groups. These reactions include esterification, where the hydroxyl groups react with carboxylic acids to form esters, and oxidation reactions, which can alter the compound's reactivity and biological activity.
Physical Properties Analysis
The physical properties of this compound, such as solubility, melting point, and crystallinity, are influenced by its molecular structure. These compounds are generally insoluble in water but can dissolve in organic solvents, which is important for their extraction and purification from natural sources.
Chemical Properties Analysis
This compound exhibits a range of chemical properties due to its complex structure. It is acidic due to the presence of carboxylic acid groups and has potential antioxidative properties owing to its phenolic constituents. These chemical characteristics contribute to its bioactivity and potential therapeutic applications.
For detailed study and further reading, please refer to the following sources:
- A study on the metabolites of the Polyporaceae, focusing on novel conjugates of polyporenic acid A from Piptoporus betulinus, which shares similarities with this compound in terms of biosynthesis and chemical properties (Bryce, Campbell, & Mccorkindale, 1967).
- Research on a new triterpenic acid from wood-rotting fungi, providing insights into the structural and chemical diversity of these compounds, which is relevant to understanding this compound (Thappa, Agarwal, Dhar, & Atal, 1981).
Scientific Research Applications
Anti-Inflammatory Properties
Polyporenic acid C, isolated from the fruiting bodies of Piptoporus betulinus, has demonstrated anti-inflammatory properties. Kamo et al. (2003) reported that this compound, along with other lanostane-type triterpene acids from Piptoporus betulinus, significantly suppressed 12-O-tetradecanoylphorbol-13-acetate (TPA)-induced edema in mouse ears, showcasing its potential as an anti-inflammatory agent (Kamo et al., 2003).
Inhibitory Effects on Osteoclastogenesis
This compound was also found to exhibit inhibitory effects on receptor activator of nuclear factor-kappaB ligand-induced osteoclastogenesis. This was discovered in a study where the compound, among others, was isolated from Antrodia heteromorpha culture medium. This finding suggests its potential application in bone health and disorders related to excessive osteoclast activity (Kwon et al., 2016).
Cytotoxic Activity
In the context of cancer research, this compound has shown cytotoxic activity. Zhou et al. (2008) isolated a variant of this compound, namely 29-hydroxythis compound, from Poria cocos, along with other compounds. These compounds exhibited significant cytotoxicity against human lung cancer cell line A549 and human prostate cancer cell line DU145, indicating their potential use in cancer therapeutics (Zhou et al., 2008).
Absorption and Bioavailability
The absorption characteristics of this compound are also of interest, particularly in the context of drug delivery and bioavailability. A study by Zheng and Yang (2008) on the absorption of triterpenoid compounds from Poria cocos, including this compound, in human intestinal epithelial cells, provides insights into its transport and potential bioavailability in human systems (Zheng & Yang, 2008).
Mechanism of Action
Polyporenic acid C, also known as UNII-8EC05EK77F, is a lanostane-type triterpenoid isolated from P. cocos . This compound has been studied for its various biological activities, including its potential role in inducing cell apoptosis .
Target of Action
This compound primarily targets acetylcholinesterase (AChE) . AChE is an enzyme that catalyzes the breakdown of acetylcholine, a neurotransmitter, in the synaptic cleft. By inhibiting AChE, this compound increases the concentration of acetylcholine in the synaptic cleft, enhancing cholinergic transmission .
Mode of Action
This compound acts as a non-competitive inhibitor of AChE . This means it binds to a site on the AChE enzyme other than the active site, altering the enzyme’s conformation and reducing its activity . This results in an increased concentration of acetylcholine in the synaptic cleft .
Biochemical Pathways
This compound affects the cholinergic pathway by inhibiting AChE . This leads to an increase in acetylcholine levels, enhancing cholinergic transmission. This mechanism is particularly relevant in the context of Alzheimer’s disease, where there is a deficiency of acetylcholine .
Result of Action
By inhibiting AChE and enhancing cholinergic transmission, this compound may have potential therapeutic effects in conditions such as Alzheimer’s disease . Additionally, it has been found to induce cell apoptosis through the death receptor-mediated apoptotic pathway without the involvement of the mitochondria . This suggests a potential role for this compound in cancer therapy .
Safety and Hazards
properties
IUPAC Name |
(2R)-2-[(5R,10S,13R,14R,16R,17R)-16-hydroxy-4,4,10,13,14-pentamethyl-3-oxo-1,2,5,6,12,15,16,17-octahydrocyclopenta[a]phenanthren-17-yl]-6-methyl-5-methylideneheptanoic acid | |
---|---|---|
Source | PubChem | |
URL | https://pubchem.ncbi.nlm.nih.gov | |
Description | Data deposited in or computed by PubChem | |
InChI |
InChI=1S/C31H46O4/c1-18(2)19(3)9-10-20(27(34)35)26-23(32)17-31(8)22-11-12-24-28(4,5)25(33)14-15-29(24,6)21(22)13-16-30(26,31)7/h11,13,18,20,23-24,26,32H,3,9-10,12,14-17H2,1-2,4-8H3,(H,34,35)/t20-,23-,24+,26+,29-,30-,31+/m1/s1 | |
Source | PubChem | |
URL | https://pubchem.ncbi.nlm.nih.gov | |
Description | Data deposited in or computed by PubChem | |
InChI Key |
KPKYWYZPIVAHKU-WMNQUVFJSA-N | |
Source | PubChem | |
URL | https://pubchem.ncbi.nlm.nih.gov | |
Description | Data deposited in or computed by PubChem | |
Canonical SMILES |
CC(C)C(=C)CCC(C1C(CC2(C1(CC=C3C2=CCC4C3(CCC(=O)C4(C)C)C)C)C)O)C(=O)O | |
Source | PubChem | |
URL | https://pubchem.ncbi.nlm.nih.gov | |
Description | Data deposited in or computed by PubChem | |
Isomeric SMILES |
CC(C)C(=C)CC[C@H]([C@H]1[C@@H](C[C@@]2([C@@]1(CC=C3C2=CC[C@@H]4[C@@]3(CCC(=O)C4(C)C)C)C)C)O)C(=O)O | |
Source | PubChem | |
URL | https://pubchem.ncbi.nlm.nih.gov | |
Description | Data deposited in or computed by PubChem | |
Molecular Formula |
C31H46O4 | |
Source | PubChem | |
URL | https://pubchem.ncbi.nlm.nih.gov | |
Description | Data deposited in or computed by PubChem | |
Molecular Weight |
482.7 g/mol | |
Source | PubChem | |
URL | https://pubchem.ncbi.nlm.nih.gov | |
Description | Data deposited in or computed by PubChem | |
CAS RN |
465-18-9 | |
Record name | Polyporenic acid C | |
Source | ChemIDplus | |
URL | https://pubchem.ncbi.nlm.nih.gov/substance/?source=chemidplus&sourceid=0000465189 | |
Description | ChemIDplus is a free, web search system that provides access to the structure and nomenclature authority files used for the identification of chemical substances cited in National Library of Medicine (NLM) databases, including the TOXNET system. | |
Record name | POLYPORENIC ACID C | |
Source | FDA Global Substance Registration System (GSRS) | |
URL | https://gsrs.ncats.nih.gov/ginas/app/beta/substances/8EC05EK77F | |
Description | The FDA Global Substance Registration System (GSRS) enables the efficient and accurate exchange of information on what substances are in regulated products. Instead of relying on names, which vary across regulatory domains, countries, and regions, the GSRS knowledge base makes it possible for substances to be defined by standardized, scientific descriptions. | |
Explanation | Unless otherwise noted, the contents of the FDA website (www.fda.gov), both text and graphics, are not copyrighted. They are in the public domain and may be republished, reprinted and otherwise used freely by anyone without the need to obtain permission from FDA. Credit to the U.S. Food and Drug Administration as the source is appreciated but not required. | |
Retrosynthesis Analysis
AI-Powered Synthesis Planning: Our tool employs the Template_relevance Pistachio, Template_relevance Bkms_metabolic, Template_relevance Pistachio_ringbreaker, Template_relevance Reaxys, Template_relevance Reaxys_biocatalysis model, leveraging a vast database of chemical reactions to predict feasible synthetic routes.
One-Step Synthesis Focus: Specifically designed for one-step synthesis, it provides concise and direct routes for your target compounds, streamlining the synthesis process.
Accurate Predictions: Utilizing the extensive PISTACHIO, BKMS_METABOLIC, PISTACHIO_RINGBREAKER, REAXYS, REAXYS_BIOCATALYSIS database, our tool offers high-accuracy predictions, reflecting the latest in chemical research and data.
Strategy Settings
Precursor scoring | Relevance Heuristic |
---|---|
Min. plausibility | 0.01 |
Model | Template_relevance |
Template Set | Pistachio/Bkms_metabolic/Pistachio_ringbreaker/Reaxys/Reaxys_biocatalysis |
Top-N result to add to graph | 6 |
Feasible Synthetic Routes
Q & A
Q1: What are the known biological activities of Polyporenic acid C (PPAC)?
A1: PPAC has been shown to exhibit various biological activities, including:
- Cytotoxicity against cancer cells: PPAC has demonstrated cytotoxicity against various cancer cell lines, including lung cancer (A549), prostate cancer (DU145), and pancreatic cancer (Panc-1, MiaPaca-2, AsPc-1 and BxPc-3). [, , ]
- Inhibition of nitric oxide (NO) production: PPAC can reduce NO production in lipopolysaccharide (LPS)-induced RAW 264.7 cells, potentially through the blockade of the activator protein-1 (AP-1) signaling pathway. []
- Inhibition of matrix metalloproteinase-7 (MMP-7): PPAC can suppress the invasive behavior of cancer cells, potentially by downregulating MMP-7 expression. []
- Induction of apoptosis: PPAC can induce apoptosis in cancer cells, likely through the death receptor-mediated apoptotic pathway involving caspase-8 activation. []
- Potential RXR agonistic activity: Research suggests PPAC may act as a retinoid X receptor (RXR) agonist, inducing differentiation in HL-60 leukemia cells and potentially contributing to anti-cancer effects. []
- Inhibition of bile acid uptake transporters: PPAC inhibits the function of apical sodium-dependent bile acid transporter (ASBT) and sodium/taurocholate cotransporting polypeptide (NTCP), suggesting a potential mechanism for its hypolipidemic effect. []
Q2: How does PPAC induce apoptosis in cancer cells?
A: Research suggests PPAC induces apoptosis in A549 lung cancer cells through the death receptor-mediated apoptotic pathway. This involves the activation of caspase-8, leading to the direct cleavage of executioner caspases, without involving the mitochondria. [] This is in contrast to pachymic acid, another lanostane-type triterpenoid, which disrupts mitochondrial membrane potential and activates caspase-9. [] Additionally, PPAC treatment suppressed the PI3-kinase/Akt signaling pathway and enhanced p53 activation, potentially contributing to apoptosis induction. []
Q3: How does PPAC affect bile acid metabolism?
A: PPAC has been identified as a competitive inhibitor of both ASBT and NTCP, two key transporters involved in the enterohepatic circulation of bile acids. [] By inhibiting these transporters, PPAC could potentially disrupt bile acid reabsorption, leading to increased bile acid excretion and potentially contributing to a hypolipidemic effect. []
Q4: What is the molecular formula and weight of this compound?
A: The molecular formula of PPAC is C30H48O4, and its molecular weight is 472.69 g/mol. [, , ]
Q5: What spectroscopic techniques are commonly used to characterize PPAC?
A: Various spectroscopic techniques are employed to elucidate the structure of PPAC, including infrared (IR) spectroscopy, ultraviolet-visible (UV-Vis) spectroscopy, nuclear magnetic resonance (NMR) spectroscopy (1H NMR, 13C NMR, COSY, NOESY, HSQC, HMBC), and mass spectrometry (MS) techniques such as electrospray ionization time-of-flight mass spectrometry (ESI-TOF-MS) and high-resolution electrospray ionization mass spectrometry (HRESI-MS). [, , , , ]
Q6: What is the main natural source of this compound?
A: PPAC is primarily isolated from the sclerotium of the fungus Poria cocos (Schw.) Wolf, also known as Wolfiporia extensa (Peck) Ginns, which is widely used in traditional Chinese medicine. [, , , , , , , , , , , ]
Q7: Can PPAC be produced by methods other than extraction from Poria cocos?
A: While PPAC is typically isolated from Poria cocos, research suggests it can also be produced through submerged cultivation of Poria cocos mycelium. This method has shown potential for higher yields of PPAC compared to traditional cultivation methods. []
Q8: How do the levels of PPAC vary in different parts of the Poria cocos fungus?
A: Studies have shown that the surface layer of Poria cocos sclerotium generally contains the highest levels of PPAC compared to other parts of the fungus. [, ] This finding suggests that focusing on the surface layer could be beneficial for maximizing PPAC extraction yields.
Q9: What analytical techniques are commonly employed for the quantification of PPAC?
A: High-performance liquid chromatography (HPLC) coupled with various detection methods, such as ultraviolet (UV) detectors and mass spectrometry (MS), are widely used for the quantification of PPAC in complex mixtures and biological samples. [, , , , , , , , ]
Q10: What are some challenges associated with the analysis of PPAC?
A: The analysis of PPAC can be challenging due to the complexity of the Poria cocos matrix, the presence of structurally similar compounds, and the potential for degradation during sample preparation and analysis. [] Therefore, developing robust and validated analytical methods is crucial for accurate quantification and quality control of PPAC.
Disclaimer and Information on In-Vitro Research Products
Please be aware that all articles and product information presented on BenchChem are intended solely for informational purposes. The products available for purchase on BenchChem are specifically designed for in-vitro studies, which are conducted outside of living organisms. In-vitro studies, derived from the Latin term "in glass," involve experiments performed in controlled laboratory settings using cells or tissues. It is important to note that these products are not categorized as medicines or drugs, and they have not received approval from the FDA for the prevention, treatment, or cure of any medical condition, ailment, or disease. We must emphasize that any form of bodily introduction of these products into humans or animals is strictly prohibited by law. It is essential to adhere to these guidelines to ensure compliance with legal and ethical standards in research and experimentation.