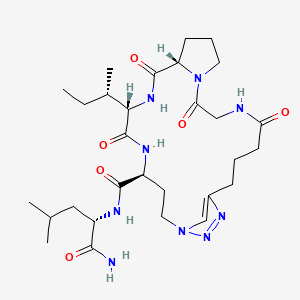
KRAS inhibitor-11
- Click on QUICK INQUIRY to receive a quote from our team of experts.
- With the quality product at a COMPETITIVE price, you can focus more on your research.
Overview
Description
KRAS inhibitor-11 is a small molecule designed to target and inhibit the activity of the KRAS protein, which is a member of the RAS family of GTPases. KRAS mutations are among the most common oncogenic drivers in human cancers, particularly in non-small cell lung cancer, colorectal cancer, and pancreatic cancer. The development of KRAS inhibitors represents a significant advancement in cancer therapeutics, as KRAS has long been considered an “undruggable” target due to its high affinity for GTP and the lack of suitable binding pockets for small molecules .
Preparation Methods
Synthetic Routes and Reaction Conditions
The synthesis of KRAS inhibitor-11 typically involves multiple steps, including the formation of key intermediates and the final coupling reactions. The synthetic route may include:
Formation of the Core Structure: This step involves the construction of the core scaffold of the molecule through a series of condensation and cyclization reactions.
Functional Group Modifications: Introduction of various functional groups to enhance the binding affinity and specificity of the inhibitor. This may involve reactions such as halogenation, alkylation, and acylation.
Final Coupling Reactions: The final step involves coupling the core structure with specific side chains or moieties that are critical for the inhibitor’s activity.
Industrial Production Methods
Industrial production of this compound involves scaling up the synthetic route to produce the compound in large quantities. This requires optimization of reaction conditions, purification processes, and quality control measures to ensure the consistency and purity of the final product .
Chemical Reactions Analysis
Types of Reactions
KRAS inhibitor-11 undergoes various chemical reactions, including:
Oxidation: The compound can be oxidized to form different oxidation states, which may affect its activity and stability.
Reduction: Reduction reactions can modify the functional groups on the inhibitor, potentially altering its binding properties.
Substitution: Substitution reactions, such as nucleophilic or electrophilic substitution, can introduce new functional groups or replace existing ones.
Common Reagents and Conditions
Common reagents used in these reactions include oxidizing agents (e.g., hydrogen peroxide), reducing agents (e.g., sodium borohydride), and various nucleophiles and electrophiles. Reaction conditions such as temperature, solvent, and pH are carefully controlled to achieve the desired transformations .
Major Products
The major products formed from these reactions include modified versions of this compound with different functional groups or oxidation states. These modifications can impact the inhibitor’s efficacy and pharmacokinetic properties .
Scientific Research Applications
KRAS inhibitor-11 has a wide range of scientific research applications, including:
Chemistry: Used as a tool compound to study the structure-activity relationships of KRAS inhibitors and to develop new analogs with improved properties.
Biology: Employed in cellular and molecular biology studies to investigate the role of KRAS in cell signaling, proliferation, and apoptosis.
Medicine: Utilized in preclinical and clinical studies to evaluate its therapeutic potential in treating KRAS-driven cancers. It is also used to study resistance mechanisms and to develop combination therapies.
Industry: Applied in the pharmaceutical industry for drug discovery and development, particularly in the design of targeted cancer therapies .
Mechanism of Action
KRAS inhibitor-11 exerts its effects by binding to the KRAS protein and inhibiting its activity. The inhibitor targets the GTP-binding pocket of KRAS, preventing the protein from switching to its active GTP-bound state. This inhibition disrupts the downstream signaling pathways, such as the RAF-MEK-ERK and PI3K-AKT pathways, which are critical for cell proliferation and survival. By blocking these pathways, this compound induces apoptosis and inhibits the growth of KRAS-driven tumors .
Comparison with Similar Compounds
KRAS inhibitor-11 can be compared with other KRAS inhibitors, such as sotorasib and adagrasib. While all these inhibitors target the KRAS protein, they differ in their binding mechanisms, specificity, and efficacy. For example:
Sotorasib: Targets the G12C mutant of KRAS and has shown clinical efficacy in non-small cell lung cancer.
Adagrasib: Also targets the G12C mutant but has a different binding profile and pharmacokinetic properties.
Pan-KRAS Inhibitors: These inhibitors target multiple KRAS isoforms and have broader applications but may have different safety and efficacy profiles .
This compound is unique in its specific binding mechanism and its potential to overcome resistance mechanisms observed with other inhibitors. Its development represents a significant advancement in the field of targeted cancer therapy .
Properties
Molecular Formula |
C29H47N9O6 |
---|---|
Molecular Weight |
617.7 g/mol |
IUPAC Name |
(13S,16S,19S)-N-[(2S)-1-amino-4-methyl-1-oxopentan-2-yl]-16-[(2S)-butan-2-yl]-5,8,14,17-tetraoxo-6,9,15,18,22,23,24-heptazatricyclo[20.2.1.09,13]pentacosa-1(25),23-diene-19-carboxamide |
InChI |
InChI=1S/C29H47N9O6/c1-5-18(4)25-29(44)32-20(27(42)33-21(26(30)41)14-17(2)3)11-13-37-16-19(35-36-37)8-6-10-23(39)31-15-24(40)38-12-7-9-22(38)28(43)34-25/h16-18,20-22,25H,5-15H2,1-4H3,(H2,30,41)(H,31,39)(H,32,44)(H,33,42)(H,34,43)/t18-,20-,21-,22-,25-/m0/s1 |
InChI Key |
ZTEFZLXCKMSXDP-HLPFYSDJSA-N |
Isomeric SMILES |
CC[C@H](C)[C@H]1C(=O)N[C@@H](CCN2C=C(CCCC(=O)NCC(=O)N3CCC[C@H]3C(=O)N1)N=N2)C(=O)N[C@@H](CC(C)C)C(=O)N |
Canonical SMILES |
CCC(C)C1C(=O)NC(CCN2C=C(CCCC(=O)NCC(=O)N3CCCC3C(=O)N1)N=N2)C(=O)NC(CC(C)C)C(=O)N |
Origin of Product |
United States |
Disclaimer and Information on In-Vitro Research Products
Please be aware that all articles and product information presented on BenchChem are intended solely for informational purposes. The products available for purchase on BenchChem are specifically designed for in-vitro studies, which are conducted outside of living organisms. In-vitro studies, derived from the Latin term "in glass," involve experiments performed in controlled laboratory settings using cells or tissues. It is important to note that these products are not categorized as medicines or drugs, and they have not received approval from the FDA for the prevention, treatment, or cure of any medical condition, ailment, or disease. We must emphasize that any form of bodily introduction of these products into humans or animals is strictly prohibited by law. It is essential to adhere to these guidelines to ensure compliance with legal and ethical standards in research and experimentation.