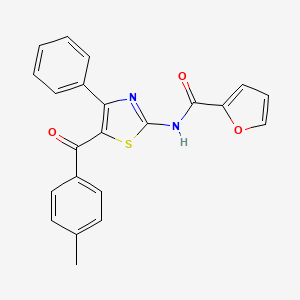
A1/A3 AR antagonist 2
- Click on QUICK INQUIRY to receive a quote from our team of experts.
- With the quality product at a COMPETITIVE price, you can focus more on your research.
Overview
Description
A1/A3 adenosine receptor antagonist 2 is a compound that targets both A1 and A3 adenosine receptors. These receptors are part of the G protein-coupled receptor family and are involved in various physiological processes, including cardiovascular function, neurotransmission, and immune response. Antagonists of these receptors have potential therapeutic applications in treating conditions such as heart failure, asthma, and certain types of cancer .
Preparation Methods
Synthetic Routes and Reaction Conditions
The synthesis of A1/A3 adenosine receptor antagonist 2 typically involves the use of 1,2,4-triazole derivatives. The synthetic route includes the following steps:
Formation of the 1,2,4-triazole scaffold: This is achieved through the cyclization of appropriate precursors under acidic or basic conditions.
Functionalization of the triazole ring: Introduction of various substituents at specific positions on the triazole ring to enhance binding affinity and selectivity for A1 and A3 receptors.
Purification and characterization: The final compound is purified using techniques such as column chromatography and characterized using spectroscopic methods like NMR and mass spectrometry.
Industrial Production Methods
Industrial production of A1/A3 adenosine receptor antagonist 2 involves scaling up the synthetic route described above. This includes optimizing reaction conditions to maximize yield and purity, as well as implementing quality control measures to ensure consistency between batches. Techniques such as high-performance liquid chromatography (HPLC) are used for purification, and automated synthesis platforms may be employed to streamline the process .
Chemical Reactions Analysis
Types of Reactions
A1/A3 adenosine receptor antagonist 2 undergoes various chemical reactions, including:
Oxidation: The compound can be oxidized using reagents like hydrogen peroxide or potassium permanganate.
Reduction: Reduction reactions can be carried out using agents such as sodium borohydride or lithium aluminum hydride.
Substitution: The compound can undergo nucleophilic or electrophilic substitution reactions, depending on the functional groups present.
Common Reagents and Conditions
Oxidation: Hydrogen peroxide, potassium permanganate, and other oxidizing agents.
Reduction: Sodium borohydride, lithium aluminum hydride, and other reducing agents.
Substitution: Nucleophiles like amines or thiols, and electrophiles like alkyl halides.
Major Products Formed
The major products formed from these reactions depend on the specific reagents and conditions used. For example, oxidation may yield hydroxylated derivatives, while reduction may produce deoxygenated compounds.
Scientific Research Applications
A1/A3 adenosine receptor antagonist 2 has a wide range of scientific research applications:
Chemistry: Used as a tool compound to study the structure-activity relationships of adenosine receptor antagonists.
Biology: Employed in research to understand the physiological roles of A1 and A3 adenosine receptors.
Medicine: Investigated for its potential therapeutic applications in treating cardiovascular diseases, asthma, and cancer.
Industry: Utilized in the development of new drugs targeting adenosine receptors .
Mechanism of Action
A1/A3 adenosine receptor antagonist 2 exerts its effects by binding to the A1 and A3 adenosine receptors, thereby blocking the action of endogenous adenosine. This inhibition prevents the activation of downstream signaling pathways, which can lead to various physiological effects. For example, blocking A1 receptors can reduce heart rate and improve cardiac function, while blocking A3 receptors can inhibit tumor growth and reduce inflammation .
Comparison with Similar Compounds
Similar Compounds
KW3902: A selective A1 adenosine receptor antagonist.
BG9928: Another selective A1 adenosine receptor antagonist.
SLV320: A selective A1 adenosine receptor antagonist with renal protective effects.
Uniqueness
A1/A3 adenosine receptor antagonist 2 is unique in its ability to target both A1 and A3 adenosine receptors simultaneously. This dual targeting can provide a broader range of therapeutic effects compared to compounds that selectively target only one receptor subtype. For example, it can offer combined benefits in treating cardiovascular diseases and cancer .
Biological Activity
Adenosine receptors (ARs) are a class of G protein-coupled receptors that play critical roles in various physiological processes. Among them, A1 and A3 receptors are particularly significant due to their involvement in numerous pathophysiological conditions, including inflammation, cancer, and cardiovascular diseases. The compound known as A1/A3 AR antagonist 2 has garnered attention for its potential therapeutic applications. This article explores the biological activity of this compound, backed by research findings, data tables, and case studies.
Overview of Adenosine Receptors
The adenosine receptor family comprises four subtypes: A1, A2A, A2B, and A3. Each subtype has distinct signaling pathways and physiological roles:
- A1 Receptor (A1R) : Primarily coupled to Gi/o proteins, inhibiting adenylate cyclase and decreasing cAMP levels.
- A3 Receptor (A3R) : Shares similar coupling with Gi/o proteins but is involved in modulating inflammatory responses and cancer cell proliferation.
Structure-Activity Relationship (SAR)
Research has shown that modifications to the chemical structure of AR antagonists can significantly affect their binding affinity and selectivity. For instance, the compound 1-methyl-3-phenyl-7-benzylaminopyrazolo[3,4-d]pyridazine was identified as a lead compound with high affinity for both A1R (21 nM) and A3R (55 nM), while demonstrating negligible activity against the A2BR subtype (<2 μM) .
Table 1: Affinities of Selected Compounds for Adenosine Receptors
Compound | A1R Affinity (nM) | A3R Affinity (nM) | A2BR Affinity (μM) |
---|---|---|---|
10b | 21 | 55 | <2 |
15b | >100 | >100 | >10 |
12 | 14 | 29 | >50 |
The mechanism by which this compound exerts its effects involves competitive inhibition at the receptor sites. Studies indicate that this compound can effectively block the action of endogenous adenosine at both the A1 and A3 receptors. This blockade leads to altered intracellular signaling pathways that can modulate inflammatory responses and inhibit tumor growth .
Inflammatory Diseases
In a study focusing on rheumatoid arthritis models, the application of A3R antagonists demonstrated a marked reduction in inflammatory markers, indicating their potential use in treating inflammatory diseases . The antagonists were shown to inhibit the proliferation of immune cells responsible for inflammation.
Cancer Research
Another significant area of research involves the role of A3R antagonists in cancer therapy. In vitro studies on prostate cancer cell lines indicated that certain compounds could reduce cell viability significantly. For example, compound 12 exhibited a GI50 value of 14 µM, demonstrating potent cytostatic activity .
Table 2: Cytotoxic Effects of Selected Compounds on Prostate Cancer Cells
Compound | GI50 (µM) | TGI (µM) | LC50 (µM) |
---|---|---|---|
Cl-IB-MECA | 10 | 20 | 30 |
12 | 14 | 29 | 59 |
Properties
Molecular Formula |
C22H16N2O3S |
---|---|
Molecular Weight |
388.4 g/mol |
IUPAC Name |
N-[5-(4-methylbenzoyl)-4-phenyl-1,3-thiazol-2-yl]furan-2-carboxamide |
InChI |
InChI=1S/C22H16N2O3S/c1-14-9-11-16(12-10-14)19(25)20-18(15-6-3-2-4-7-15)23-22(28-20)24-21(26)17-8-5-13-27-17/h2-13H,1H3,(H,23,24,26) |
InChI Key |
NSGJWVVFFNBRGB-UHFFFAOYSA-N |
Canonical SMILES |
CC1=CC=C(C=C1)C(=O)C2=C(N=C(S2)NC(=O)C3=CC=CO3)C4=CC=CC=C4 |
Origin of Product |
United States |
Disclaimer and Information on In-Vitro Research Products
Please be aware that all articles and product information presented on BenchChem are intended solely for informational purposes. The products available for purchase on BenchChem are specifically designed for in-vitro studies, which are conducted outside of living organisms. In-vitro studies, derived from the Latin term "in glass," involve experiments performed in controlled laboratory settings using cells or tissues. It is important to note that these products are not categorized as medicines or drugs, and they have not received approval from the FDA for the prevention, treatment, or cure of any medical condition, ailment, or disease. We must emphasize that any form of bodily introduction of these products into humans or animals is strictly prohibited by law. It is essential to adhere to these guidelines to ensure compliance with legal and ethical standards in research and experimentation.