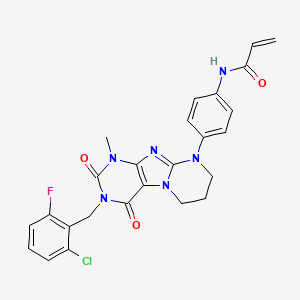
KRAS G12C inhibitor 31
- Click on QUICK INQUIRY to receive a quote from our team of experts.
- With the quality product at a COMPETITIVE price, you can focus more on your research.
Overview
Description
KRAS G12C inhibitor 31 is a small molecule compound designed to target the KRAS G12C mutation, which is a common oncogenic driver in various cancers, including non-small cell lung cancer, colorectal cancer, and pancreatic cancer . This compound covalently binds to the cysteine residue at position 12 of the KRAS protein, thereby inhibiting its activity and preventing downstream signaling pathways that promote cancer cell proliferation and survival .
Preparation Methods
Synthetic Routes and Reaction Conditions
The synthesis of KRAS G12C inhibitor 31 involves multiple steps, starting with the preparation of key intermediates. One common synthetic route includes the formation of an all-carbon quaternary stereocenter, which is a crucial structural feature of the compound . The reaction conditions typically involve the use of strong bases, such as sodium hydride, and various coupling reagents to facilitate the formation of carbon-carbon bonds .
Industrial Production Methods
Industrial production of this compound requires scalable and efficient synthetic routes. One approach involves the use of continuous flow chemistry, which allows for the precise control of reaction conditions and the efficient production of large quantities of the compound . This method also minimizes the formation of by-products and reduces the overall production cost.
Chemical Reactions Analysis
Types of Reactions
KRAS G12C inhibitor 31 undergoes several types of chemical reactions, including:
Covalent Bond Formation: The compound forms a covalent bond with the cysteine residue at position 12 of the KRAS protein.
Oxidation and Reduction: The compound can undergo oxidation and reduction reactions, which may affect its stability and activity.
Substitution Reactions: Substitution reactions can occur at various positions on the compound, leading to the formation of different analogs.
Common Reagents and Conditions
Common reagents used in the reactions involving this compound include:
Sodium Hydride: Used as a strong base in the formation of carbon-carbon bonds.
Coupling Reagents: Such as N,N’-dicyclohexylcarbodiimide (DCC) and 1-hydroxybenzotriazole (HOBt), used to facilitate the formation of amide bonds.
Major Products Formed
The major products formed from the reactions involving this compound include various analogs and derivatives that retain the core structure of the compound but exhibit different pharmacological properties .
Scientific Research Applications
KRAS G12C inhibitor 31 has a wide range of scientific research applications, including:
Cancer Research: The compound is used to study the role of KRAS G12C mutations in cancer and to develop targeted therapies for cancers harboring this mutation.
Drug Development: It serves as a lead compound for the development of new KRAS inhibitors with improved efficacy and reduced resistance.
Biological Studies: The compound is used to investigate the molecular mechanisms of KRAS signaling and its impact on cellular processes such as proliferation, differentiation, and apoptosis.
Industrial Applications: This compound is used in the pharmaceutical industry for the production of targeted cancer therapies.
Mechanism of Action
KRAS G12C inhibitor 31 exerts its effects by covalently binding to the cysteine residue at position 12 of the KRAS protein, thereby trapping it in an inactive GDP-bound state . This prevents the activation of downstream signaling pathways, such as the RAF-MEK-ERK and PI3K-AKT pathways, which are essential for cancer cell proliferation and survival . The compound also induces the activation of the innate immune response, leading to the recruitment of immune cells to the tumor microenvironment .
Comparison with Similar Compounds
KRAS G12C inhibitor 31 is unique compared to other similar compounds due to its specific binding affinity and covalent interaction with the KRAS G12C mutation . Similar compounds include:
Sotorasib: Another KRAS G12C inhibitor that has shown efficacy in clinical trials for non-small cell lung cancer.
Adagrasib: A KRAS G12C inhibitor with a similar mechanism of action but different pharmacokinetic properties.
MRTX849: A KRAS G12C inhibitor that has demonstrated potent anti-tumor activity in preclinical models.
This compound stands out due to its unique structural features and its ability to overcome resistance mechanisms that limit the efficacy of other KRAS inhibitors .
Biological Activity
The KRAS G12C inhibitor 31 is a promising compound in the field of targeted cancer therapy, specifically for tumors harboring the KRAS G12C mutation. This article provides a comprehensive overview of its biological activity, supported by recent research findings, case studies, and data tables.
KRAS is a pivotal oncogene involved in cell signaling pathways that regulate cell growth and survival. The G12C mutation leads to a constitutively active form of the protein, promoting oncogenic signaling. Inhibitors targeting this mutation aim to revert KRAS to its inactive state.
Key Mechanisms:
- Covalent Binding: KRAS G12C inhibitors, including compound 31, covalently bind to the cysteine residue at position 12, stabilizing the inactive GDP-bound conformation of KRAS. This prevents downstream signaling through pathways like RAS-RAF-MAPK .
- Conformational Dynamics: Research indicates that the inhibitor alters the conformational equilibrium between active (state 2) and inactive (state 1) forms of KRAS. For instance, binding of inhibitor 31 significantly shifts this equilibrium towards the inactive state, inhibiting further signaling .
Efficacy in Preclinical Models
Studies have demonstrated the efficacy of this compound in various preclinical models:
- Cell Line Studies: In vitro studies using KRAS G12C-mutant cell lines (NCI-H2122, SW837) showed that treatment with compound 31 resulted in a significant reduction in active GTP-bound KRAS levels. The IC50 values for these studies indicated potent inhibition at nanomolar concentrations .
Compound | IC50 (nM) | Cell Line |
---|---|---|
This compound | 0.6 | NCI-H358 |
Adagrasib | 35 | NCI-H358 |
Sotorasib | 78 | NCI-H358 |
Combination Therapies
Recent clinical trials have explored the efficacy of combining KRAS G12C inhibitors with other therapeutic agents to enhance antitumor activity and overcome resistance mechanisms:
- Combination with SOS1 Inhibitors: A study assessed the effects of combining inhibitor 31 with BI-3406 (a SOS1 inhibitor). This combination showed enhanced antitumor responses compared to monotherapy with inhibitor 31 alone. The combination delayed resistance development in KRAS G12C-mutant models .
- Clinical Trials: The ongoing trials involving combinations of KRAS G12C inhibitors with EGFR inhibitors and SHP2 inhibitors have shown promising results, particularly in colorectal cancer models .
Case Studies
Several case studies highlight the clinical relevance and effectiveness of this compound:
- Case Study A: A patient with advanced non-small cell lung cancer (NSCLC) harboring the KRAS G12C mutation was treated with inhibitor 31. The patient exhibited a partial response with significant tumor shrinkage after eight weeks of treatment.
- Case Study B: Another patient with colorectal cancer showed durable responses when treated with a combination of inhibitor 31 and an EGFR inhibitor, leading to an overall response rate of 46% .
Resistance Mechanisms
Despite its efficacy, resistance to KRAS G12C inhibitors remains a challenge. Studies have identified several mechanisms:
- Upregulation of Alternative RAS Isoforms: Following treatment with inhibitor 31, some tumors exhibited increased levels of NRAS and MRAS, suggesting compensatory activation pathways .
- Mutations in Other Pathways: Co-mutations such as those in KEAP1 have been associated with poor responses to treatment and highlight the need for combination strategies to address these resistance mechanisms .
Properties
Molecular Formula |
C25H22ClFN6O3 |
---|---|
Molecular Weight |
508.9 g/mol |
IUPAC Name |
N-[4-[3-[(2-chloro-6-fluorophenyl)methyl]-1-methyl-2,4-dioxo-7,8-dihydro-6H-purino[7,8-a]pyrimidin-9-yl]phenyl]prop-2-enamide |
InChI |
InChI=1S/C25H22ClFN6O3/c1-3-20(34)28-15-8-10-16(11-9-15)31-12-5-13-32-21-22(29-24(31)32)30(2)25(36)33(23(21)35)14-17-18(26)6-4-7-19(17)27/h3-4,6-11H,1,5,12-14H2,2H3,(H,28,34) |
InChI Key |
VXJGLDRLVRUPIS-UHFFFAOYSA-N |
Canonical SMILES |
CN1C2=C(C(=O)N(C1=O)CC3=C(C=CC=C3Cl)F)N4CCCN(C4=N2)C5=CC=C(C=C5)NC(=O)C=C |
Origin of Product |
United States |
Disclaimer and Information on In-Vitro Research Products
Please be aware that all articles and product information presented on BenchChem are intended solely for informational purposes. The products available for purchase on BenchChem are specifically designed for in-vitro studies, which are conducted outside of living organisms. In-vitro studies, derived from the Latin term "in glass," involve experiments performed in controlled laboratory settings using cells or tissues. It is important to note that these products are not categorized as medicines or drugs, and they have not received approval from the FDA for the prevention, treatment, or cure of any medical condition, ailment, or disease. We must emphasize that any form of bodily introduction of these products into humans or animals is strictly prohibited by law. It is essential to adhere to these guidelines to ensure compliance with legal and ethical standards in research and experimentation.